Information
- Author Services

Initiatives
You are accessing a machine-readable page. In order to be human-readable, please install an RSS reader.
All articles published by MDPI are made immediately available worldwide under an open access license. No special permission is required to reuse all or part of the article published by MDPI, including figures and tables. For articles published under an open access Creative Common CC BY license, any part of the article may be reused without permission provided that the original article is clearly cited. For more information, please refer to https://www.mdpi.com/openaccess .
Feature papers represent the most advanced research with significant potential for high impact in the field. A Feature Paper should be a substantial original Article that involves several techniques or approaches, provides an outlook for future research directions and describes possible research applications.
Feature papers are submitted upon individual invitation or recommendation by the scientific editors and must receive positive feedback from the reviewers.
Editor’s Choice articles are based on recommendations by the scientific editors of MDPI journals from around the world. Editors select a small number of articles recently published in the journal that they believe will be particularly interesting to readers, or important in the respective research area. The aim is to provide a snapshot of some of the most exciting work published in the various research areas of the journal.
Original Submission Date Received: .
- Active Journals
- Find a Journal
- Proceedings Series
- For Authors
- For Reviewers
- For Editors
- For Librarians
- For Publishers
- For Societies
- For Conference Organizers
- Open Access Policy
- Institutional Open Access Program
- Special Issues Guidelines
- Editorial Process
- Research and Publication Ethics
- Article Processing Charges
- Testimonials
- Preprints.org
- SciProfiles
- Encyclopedia
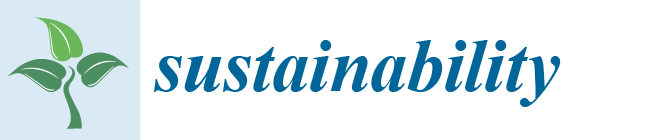
Article Menu
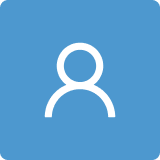
- Subscribe SciFeed
- Recommended Articles
- Google Scholar
- on Google Scholar
- Table of Contents
Find support for a specific problem in the support section of our website.
Please let us know what you think of our products and services.
Visit our dedicated information section to learn more about MDPI.
JSmol Viewer
A case study on renewable energy sources, power demand, and policies in the states of south india—development of a thermoelectric model.
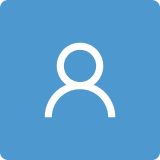
1. Introduction
1.1. background, 1.2. literature report, 1.3. objectives, 1.4. organization of the article, 2. conventional and renewable energy resources—a review, 2.1. conventional energy resources, limitations of conventional energy:.
- Eliminating coal, oil, and gas is unsafe and can cause pollution. As a result, these petrol subordinates are non-feasible.
- As we go through viably accessible wellsprings of coal, oil, and gas, removing them turns out to be all of the more genuinely, more expensive, and more unsafe.
- Burning-through oil subordinates (both for warming and as fuel for vehicles) is the guideline wellspring of ‘ozone hurting substances’, carbon dioxide, and others that impact the air and are changing the climate.
- Contamination: the significant hindrance of these regular sources is that they cause high contamination. The consumption of kindling and petroleum products brings about air contamination. This can stay away from utilizing these non-regular sources.
- Modesty: The serious issue while utilizing regular sources, particularly petroleum products is that they are expendable sources. It requires a long period of time for them to be restored and recharged. In any case, non-regular sources are inexhaustible sources that do not get depleted.
- Dangerous: non-regular energy extraction is more secure. Numerous mishaps happen while removing energy from mines.
- Significant expense: the extraction of these energy sources is exorbitant both monetarily and on earth. The expense of energy creation and extraction is a lot less for non-ordinary sources (assuming that the underlying expense of foundation is borne).
2.2. Clean Energy
- Clean energy = clean air
- Successful power energy = standard sources
- Efficient power = recyclable sources
Favorable of Clean Energy
- Clean energy provides an assortment of ecological and monetary advantages, such as providing a decrease in air contamination. A different clean energy supply likewise decreases the reliance on imported energies (and the related monetary and natural costs this brings about).
- Sustainable clean energy likewise has inborn expense investment funds, as there is no compelling reason to concentrate and move powers (for example, with oil or coal, as the assets recharge themselves normally).
- Another modern advantage of a spotless energy blend is the formation of tasks to create, fabricate and introduce the perfect energy assets of things to come.
2.3. Renewable Energy in India—A Glance
Country | Score | Recai Rank |
---|---|---|
USA | 70.7 | 1 |
INDIA | 66.2 | 2 |
CHINA | 68.7 | 3 |
Click here to enlarge figure
3. Energy Mix of Southern States
3.1. power—energy scenario in the state of andhra pradesh.
- The total capacity of the thermal power plant installed is 3410.0 MW.
- The total capacity of the Hydel power plant is 1773.6 MW.
- The total capacity of power plants using non-conventional energy resources is 405.426 MW. Thus, the overall total capacity of plants under APGENCO is 5589.0 MW.
- The total installed capacity of the Andhra Pradesh Southern Power Distribution Company Limited (APSPDCL) is 1600 MW.
- The total installed capacity under the Government of Andhra Pradesh (A.P) is 7189.0 MW.
Year | APERC Approved Peak Demand | Alternate Scenario (In Worst Case) |
---|---|---|
2019–2020 | 11,450 MW | 18,23 MW |
2020–2021 | 12,219 MW | 19,547 MW |
2021–2022 | 13,209 MW | 19,357 MW |
2022–2023 | 14,315 MW | 19,786 MW |
2023–2024 | 15,539 MW | 19,867 MW |
Current Installed Capacity of the state: 20,081 MW | ||
Current Peak Demand: 10,207 MW |
3.2. Power—Energy Scenario in the State of Tamilnadu
- To promote the use of new and renewable sources of energy (NRSE), and therefore to implement projects.
- To encourage people to participate in energy-saving initiatives,
- To promote scientific research and development of renewable sources of energy.
3.3. Energy Scenario in the State of Kerala
- Kerala State Electricity Board (KSEBL): the KSEBL generates a total power of 2246.685 MW of which the hydro power plant contributes to about 2052.00 MW, the diesel/low Sulphur heavy stock (LSHS) based power plant contributes about 159.96 MW, the wind energy based power plant contributes about 2.025 MW, and solar energy-based power plant contributes up to 32.70 MW.
- Captive Power Plant: the Captive power plant contributes a total power of about 85.7 MW of which the hydro power plant contributes about 33 MW, the solar energy-based power plant contributes about 32.70 MW, and the wind and thermal energy-based power plants contribute up to 10 MW each. Independent power producers-based power plants generate a total power of 502.83 MW of which thermal power plants contribute 359.58 MW, hydropower plants contribute about 33.00 MW, wind energy-based power plant contributes about 58.25 MW, and solar energy-based power plants contribute about 52.00 MW.
- Co-generation Power Plant: the co-generation thermal-based power plant contributes up to 10 MW. Thus, the overall installed capacity of the state is 2823.0140 MW. The installed capacity of MW as of 2021 in Kerala by the Kerala State Electricity Board (KSEBL) by various types of power stations is described in the Table 4 .
Controlled by | Type of Power Station | Total Installed Capacity (MW) |
---|---|---|
KSEBL | Hydro Power Plant | 2052.00 MW |
Diesel/LSHS | 159.96 MW | |
Wind Energy | 2.025 MW | |
Solar Energy | 32.70 MW | |
CAPTIVE | Hydro Power Plant | 33.00 MW |
Solar Energy | 32.70 MW | |
Wind Energy | 10.00 MW | |
Thermal Energy | 10.00 MW | |
IPP | Thermal Energy | 359.58 MW |
Hydro Power Plant | 33.00 MW | |
Wind Energy | 58.25 MW | |
Solar Energy | 52.00 MW | |
Co-generation | Thermal | 10.00 MW |
Total | 2823.0140 MW |
3.4. Power—Energy Scenario in the State of Karnataka
3.5. the state of odisha—power production and supply a glance, 3.6. power policies formulated in various states across south india, 3.7. gas emission from various renewable energy sources, 4. budget allocation by southern states—a comparison, 5. thermo-electric-generator (teg), 5.1. methodology and materials, 5.2. simulation study of the proposed teg model, 5.3. experimentation of the proposed teg model, 6. conclusions, author contributions, institutional review board statement, informed consent statement, conflicts of interest.
- Krishnamoorthy, R.; Udhayakumar, K.; Kannadasan, R.; Madurai Elavarasan, R.; Mihet-Popa, L. An Assessment of Onshore and Offshore Wind Energy Potential in India Using Moth Flame Optimization. Energies 2020 , 13 , 3063. [ Google Scholar ]
- Ganesan, S.; Subramaniam, U.; Ghodke, A.A.; Elavarasan, R.M.; Raju, K.; Bhaskar, S.M. Investigation on Sizing of Voltage Source for a Battery Energy Storage System in Microgrid with Renewable Energy Sources. IEEE Access 2020 , 8 , 188861–188874. [ Google Scholar ] [ CrossRef ]
- Elavarasan, R.M.; Selvamanohar, L.; Raju, K.; Vijayaraghavan, R.R.; Subburaj, R.; Nurunnabi, M.; Khan, I.A.; Afridhis, S.; Hariharan, A.; Pugazhendhi, R.; et al. A Holistic Review of the Present and Future Drivers of the Renewable Energy Mix in Maharashtra, State of India. Sustainability 2020 , 12 , 6596. [ Google Scholar ] [ CrossRef ]
- Elavarasan, R.M.; Shafiullah, G.M.; Raju, K.; Mudgal, V.; Arif, M.T.; Jamal, T.; Subramanian, S.; Balaguru, V.S.; Reddy, K.; Subramaniam, U. COVID-19: Impact analysis and recommendations for power sector operation. Appl. Energy 2020 , 279 , 115739. [ Google Scholar ] [ CrossRef ]
- Anthony, M.; Prasad, V.; Raju, K.; Alsharif, M.H.; Geem, Z.W.; Hong, J. Design of Rotor Blades for Vertical Axis Wind Turbine with Wind Flow Modifier for Low Wind Profile Areas. Sustainability 2020 , 12 , 8050. [ Google Scholar ] [ CrossRef ]
- Hameed, S.S.; Ramadoss, R.; Raju, K.; Shafiullah, G. A Framework-Based Wind Forecasting to Assess Wind Potential with Improved Grey Wolf Optimization and Support Vector Regression. Sustainability 2022 , 14 , 4235. [ Google Scholar ] [ CrossRef ]
- Patel, R.K.; Kumari, A.; Tanwar, S.; Hong, W.-C.; Sharma, R. AI-Empowered Recommender System for Re-newable Energy Harvesting in Smart Grid System. IEEE Access 2022 , 10 , 24316–24326. [ Google Scholar ] [ CrossRef ]
- Wu, C.; Zhang, X.-P.; Sterling, M. Wind power generation variations and aggregations. CSEE J. Power Energy Syst. 2022 , 8 , 17–38. [ Google Scholar ]
- Si, Y.; Chen, L.; Zhang, X.; Chen, X.; Mei, S. Capacity allocation of hybrid power system with hot dry rock ge-othermal energy, thermal storage, and PV based on game approaches. J. Mod. Power Syst. Clean Energy 2022 , 1–12. [ Google Scholar ] [ CrossRef ]
- Arief, I.S.; Aldara, D.R. Preliminary Design of Ocean Thermal Energy Conversion (OTEC) Axial Turbine for Laboratory Scale. In Proceedings of the 2018 Asian Conference on Energy, Power and Transportation Electrification (ACEPT), Singapore, 30 October–2 November 2018; pp. 1–8. [ Google Scholar ]
- Kishore, D.R.; Prasnnamba, T.J. An application of OTEC principle to thermal power plant as a co-generation plant. In Proceedings of the 2017 2nd International Conference on Communication and Electronics Systems (ICCES), Coimbatore, India, 19–20 October 2017; pp. 992–995. [ Google Scholar ]
- Li, Y.; Liu, Y.; Bai, W.; Li, B.; Xu, L. A Data-based Water-inflow Forecasting Method for Small/medium Sized Hydropower Plants in Spot Market. In Proceedings of the 2021 IEEE Sustainable Power and Energy Conference (iSPEC), Nanjing, China, 23–25 December 2021; pp. 3675–3679. [ Google Scholar ]
- De Angelis, E.; Carnevale, C.; Marcoberardino, G.D.; Turrini, E.; Volta, M. Low Emission Road Transport Scenarios: An Integrated Assessment of Energy Demand, Air Quality, GHG Emissions, and Costs. IEEE Trans. Autom. Sci. Eng. 2022 , 19 , 37–47. [ Google Scholar ] [ CrossRef ]
- Rivas, M.J.A.R.; Capuano, D.; Miranda, C. Economic and Environmental Performance of Biowaste-to-energy Technologies for Small-scale Electricity Generation. J. Mod. Power Syst. Clean Energy 2022 , 10 , 12–18. [ Google Scholar ] [ CrossRef ]
- Duarte, J.L.R.; Fan, N. Operation of a Power Grid with Embedded Networked Microgrids and Onsite Renewable Technologies. Energies 2022 , 15 , 2350. [ Google Scholar ] [ CrossRef ]
- Yue, C.-D.; Wang, I.-C.; Huang, J.-S. Feasibility of Replacing Nuclear and Fossil Fuel Energy with Offshore Wind Energy: A Case for Taiwan. Energies 2022 , 15 , 2385. [ Google Scholar ] [ CrossRef ]
- Muteri, V.; Guarino, F.; Longo, S.; Bua, L.; Cellura, M.; Testa, D.; Bonzi, M. An Innovative Photovoltaic Luminescent Solar Concentrator Window: Energy and Environ-mental Aspects. Sustainability 2022 , 14 , 4292. [ Google Scholar ] [ CrossRef ]
- Petracca, E.; Faraggiana, E.; Ghigo, A.; Sirigu, M.; Bracco, G.; Mattiazzo, G. Design and Techno-Economic Analysis of a Novel Hybrid Offshore Wind and Wave Energy System. Energies 2022 , 15 , 2739. [ Google Scholar ] [ CrossRef ]
- Liszka, D.; Krzemianowski, Z.; Węgiel, T.; Borkowski, D.; Polniak, A.; Wawrzykowski, K.; Cebula, A. Alternative Solutions for Small Hydropower Plants. Energies 2022 , 15 , 1275. [ Google Scholar ] [ CrossRef ]
- Nasab, N.M.; Islam, R.; Muttaqi, K.; Sutanto, D. Optimization of a Grid-Connected Microgrid Using Tidal and Wind Energy in Cook Strait. Fluids 2021 , 6 , 426. [ Google Scholar ] [ CrossRef ]
- Davison, N.; Gaxiola, J.B.; Gupta, D.; Garg, A.; Cockerill, T.; Tang, Y.; Yuan, X.; Ross, A. Potential Greenhouse Gas Mitigation for Converting High Moisture Food Waste into Bio-Coal from Hydrothermal Carbonisation in India, Europe and China. Energies 2022 , 15 , 1372. [ Google Scholar ] [ CrossRef ]
- Vokurka, M.; Kunz, A. Case Study of Using the Geothermal Potential of Mine Water for Central District Heating—The Rožná Deposit, Czech Republic. Sustainability 2022 , 14 , 2016. [ Google Scholar ] [ CrossRef ]
- Rahimoon, A.A.; Soomro, D.M.; Abdullah, M.N.; Soho, I.A.; Soomro, S.A.; Ali, S.N. Development of an Educational Solar Tracking Parabolic Dish Using Raspberry Pi. In Proceedings of the 2019 IEEE 6th International Conference on Engineering Technologies and Applied Sciences (ICETAS), Kuala Lumpur, Malaysia, 20–21 December 2019; pp. 1–5. [ Google Scholar ]
- Nyandang, A.N.A.; Singh, B.S.A.B. An Experimental Study of the Effect of Cooling Method in Parabolic Solar Dish Concentrator for Power Generation using Thermoelectric Generator. In Proceedings of the 2019 International UNIMAS STEM 12th Engineering Conference (EnCon), Kuching, Malaysia, 28–29 August 2019; pp. 46–51. [ Google Scholar ]
- Arifin, M.; Rajani, A.; Atmaja, T.D. Modeling and Performance Analysis of a Parallel Solar Hybrid Micro Gas Turbine. In Proceedings of the 2019 International Conference on Sustainable Energy Engineering and Application (ICSEEA), Tangerang, Indonesia, 23–24 October 2019; pp. 62–68. [ Google Scholar ]
- Benbaziz, D.E.M.; Abdellatif, H.; Ramdani, M. Conception and realization of a parabolic dish. In Proceedings of the 2019 Inter-national Conference on Advanced Systems and Emergent Technologies (IC_ASET), Hammamet, Tunisia, 19–22 March 2019; pp. 79–83. [ Google Scholar ]
- López, O.; Sáez, J.F.; Baños, A.; Arenas, A. Modeling and Control of Steam Generation with a Parabolic Dish Collector. In Proceedings of the 2019 5th International Conference on Event-Based Control, Communication, and Signal Processing (EBCCSP), Vienna, Austria, 27–29 May 2019; pp. 1–4. [ Google Scholar ]
- Toygar, M.E.; Bayram, T.; Incesu, O.; Cetin, Z.; Toygar, A. Lower construction of solarux CSP greenhouse, provides extra incomes in addition to electricity generation with new designed CSP dish mirrors. In Proceedings of the 2018 IEEE 12th International Conference on Compatibility, Power Electronics and Power Engineering (CPE-POWERENG 2018), Doha, Qatar, 10–12 April 2018; pp. 1–6. [ Google Scholar ]
- Wardhana, A.S.; Suryoatmojo, H.; Ashari, M. Design of parabolic solar concentrator to improve the optical efficiency for thermal engine generators using dual reflector Gregorian method. In Proceedings of the 2016 International Seminar on Intelligent Technology and Its Applications (ISITIA), Lombok, Indonesia, 28–30 July 2016; pp. 457–464. [ Google Scholar ]
- Available online: https://assets.ey.com/content/dam/ey-sites/ey-com/en_gl/topics/power-and-utilities/power-and-utilities-pdf/ey-recai-57-top-40-ladder.pdf (accessed on 20 May 2020).
- Ernst & Young. 2021 Renewable Energy Country Attractiveness Index (RECAI). Available online: https://www.ey.com (accessed on 20 May 2020).
- Available online: https://apgenco.gov.in/Main/page/1/65 (accessed on 20 May 2020).
- Available online: https://mercomindia.com/indias-solar-generation-up-27-yoy-q1-2022/ (accessed on 25 May 2020).
- Available online: https://aperc.gov.in/admin/upload/LFRPPP.pdf (accessed on 25 May 2020).
- Available online: https://apedb.gov.in/power-energy-sector.html (accessed on 20 May 2020).
- Available online: https://timesofindia.indiatimes.com/city/chennai/tamil-nadu-to-add-18000mw-power-generation-capacity-in-10-years/articleshow/85319621 (accessed on 23 May 2020).
- Available online: https://en.wikipedia.org/wiki/Tamil_Nadu_Generation_and_Distribution_Corporation (accessed on 20 May 2020).
- Available online: https://www.kseb.in/index.php?option=com_content&view=article&id=45&Itemid=553&lang=en (accessed on 25 May 2020).
- Kumar, A.G.; Anmol, M.; Akhil, V.S. A Strategy to Enhance Electric Vehicle Penetration Level in India. Procedia Technol. 2015 , 21 , 552–559. [ Google Scholar ] [ CrossRef ] [ Green Version ]
- Available online: https://www.statista.com/statistics/1077713/india-kerala-installed-power-capacity/ (accessed on 21 May 2020).
- Available online: https://bescom.karnataka.gov.in/new-page/Load%20Curve/en (accessed on 26 May 2020).
- Available online: https://energy.karnataka.gov.in/info-2/Power+Generation/en (accessed on 20 May 2020).
- Available online: https://powermin.gov.in/sites/default/files/uploads/joint_initiative_of_govt_of_india_and_Orissa.pdf (accessed on 24 May 2020).
- Available online: https://oredaodisha.com/ (accessed on 20 May 2020).
- Available online: http://tidco.com/wp-content/uploads/2020/04/tamil-nadu-solar-policy-2019-min.pdf (accessed on 20 May 2020).
- Available online: https://www.indiabudget.gov.in/ (accessed on 20 May 2020).
- Available online: https://nredcap.in/PDFs/Pages/AP_Solar_Power_Policy_2018.pdf (accessed on 25 May 2020).
- Available online: http://www.cbip.org/policies2019/PD_07_Dec_2018_Policies/Karnataka/2-RE%20Draft/1%20Summary%20Draft%20Karnataka%20Renewable%20Energy%20Policy%202016-22.pdf (accessed on 23 May 2020).
- Available online: https://ekiran.kseb.in/public/documents/Kerala-Solar-Power-Policy.pdf (accessed on 23 May 2020).
- Available online: https://spc.tn.gov.in/12plan_english/9-Energy.pdf (accessed on 22 May 2020).
- Shahbaz, R.; Ahmed, T.; Elavarasan, R.M.; Raju, K.; Waqas, M.; Subramaniam, U. Selective Harmonics Elimination in Multilevel Inverter Using Bio-Inspired Intelligent Algorithms. In Proceedings of the 2021 31st Aus-tralasian Universities Power Engineering Conference (AUPEC), Perth, Australia, 26–30 September 2021; pp. 1–6. [ Google Scholar ] [ CrossRef ]
- Rameshkumar, T.; Chandrasekar, P.; Kannadasan, R.; Thiyagarajan, V.; Alsharif, M.H.; Kim, J.H. Electrical and Mechanical Characteristics Assessment of Wind Turbine System Employing Acoustic Sensors and Matrix Converter. Sustainability 2022 , 14 , 4404. [ Google Scholar ] [ CrossRef ]
- Alsharif, M.H.; Kannadasan, R.; Jahid, A.; Albreem, M.A.; Nebhen, J.; Choi, B.J. Long-Term Techno-Economic Analysis of Sustainable and Zero Grid Cellular Base Station. IEEE Access 2021 , 9 , 54159–54172. [ Google Scholar ] [ CrossRef ]
- Alsharif, M.H.; Kannadasan, R.; Hassan, A.Y.; Tawfik, W.Z.; Kim, M.-K.; Khan, M.A.; Solyman, A.A.A. Optimization Analysis of Sustainable Solar Power System for Mobile Communication Systems. Comput. Mater. Contin. 2022 , 71 , 3243–3255. [ Google Scholar ] [ CrossRef ]
Total Installed Capacity of APGENCO | ||
---|---|---|
S. No. | Type of Power Plant | Total Installed Capacity (In MW) |
1 | Thermal Power Plant | 3410.0 |
2 | Hydel Power Plant | 1773.6 |
3 | Non-conventional Power Plant | 405.426 |
4 | Total | 5589.0 |
S. No. | Power Plant Based Upon Source | Total Installed Capacity in MW |
---|---|---|
1. | Hydro | 3798 MW |
2. | Thermal | 5020 MW |
3. | CGS | 4415 MW |
4. | Wind | 5095.44 MW |
5. | Co-Generation | 1731.16 MW |
6. | Mini Hydel | 903.46 MW |
7. | Bio Mass | 139.03 MW |
8. | Solar | 7505.46 MW |
9. | Captive | 992.3 MW |
10. | IPP | 1200 MW |
Total | 30,799.85 MW |
Andhra Pradesh | Karnataka | Kerala | Tamil Nadu |
---|---|---|---|
]. | ]. | ]. | ]. |
Inference of power policy of Andhra Pradesh: The power policy of Andhra Pradesh is mainly focused on encouraging the development of solar power projects for the sale of electricity, solar roof-top projects, solar parks and solar powered pumpsets. | Inference of power policy of Karnataka: The power policy of Karnataka is mainly invested on encouraging the development renewable energy projects by making the state—investment friendly. | Inference of power policy of Kerala: The power policy of Kerala is mainly focused on encouraging the installation of Solar panels for energy production and solar water heating systems for heating in large scale. | Inference of power policy of Tamil Nadu: The power policy of Tamil Nadu is mainly focused on encouraging the consumers to become a prosumer and configuring new energy meters for better monitoring of energy production and energy usage. |
S. No. | State | 2019–2020 Actuals | 2020–2021 Budget Estimates (BE) | 2020–2021 Revised Estimates (RE) | 2021–2022 BE | Annualized Change (2019–2020 to 2021–2022 BE) | Budget Provisions 2021–2022 |
---|---|---|---|---|---|---|---|
1. | Karnataka | 13,123 | 12,918 | 12,918 | 12,576 | −2% | Subsidies of Rs. 9167 crores have been allocated to Karnataka Power Transmission Corporation (KPTC) to promote renewable energy- based power production. |
2. | Kerala | 17.34 | 386.92 | 907.00 | 454.44 | 84% | Rs. 2 crore has been allotted for KSEBL. |
3. | Andhra Pradesh | 11,592.04 | 6176.14 | 6078.45 | 6438.80 | −15% | Subsidies of Rs 2568.29 lakhs have been allotted to the PTRANSCO, DISCOMS, and APGENCO. |
4. | Telangana | 7222 | 10,111 | 10,111 | 10,633 | 22% | Rs. 7665 crore has been allocated along with allied subsidies towards assistance to the Transmission Corporation of Telangana Limited (TSTRANSCO) to promote solar based power production in agricultural sector. |
5. | Tamil Nadu | 9497 | 13,118 | 17,042 | 16,020 | 30% | Rs. 7108 crore been allocated for taking over the future loss of Tamil Nadu Generation and Distribution Corporation Limited (TANDGEDCO) under UDAY scheme. |
6. | Orissa | 22.0237 | 103.4661 | 103.4665 | 52.2729 | 46% | Rs. 49.56 crore has been allocated for new and renewable energy development. |
Design Details | Specifications |
---|---|
Parabola—Diameter of the open mouth | 0.66 m |
Parabolic Concentrator—Surface area | 0.342 m |
Parabola—Height | 0.0508 m |
Concentrator—Reflectivity | 0.78 |
Focal distance | 0.48 m |
MDPI stays neutral with regard to jurisdictional claims in published maps and institutional affiliations. |
Share and Cite
Loganathan, V.; Ravikumar, D.; Kesavan, R.; Venkatesan, K.; Saminathan, R.; Kannadasan, R.; Sudhakaran, M.; Alsharif, M.H.; Geem, Z.W.; Hong, J. A Case Study on Renewable Energy Sources, Power Demand, and Policies in the States of South India—Development of a Thermoelectric Model. Sustainability 2022 , 14 , 8882. https://doi.org/10.3390/su14148882
Loganathan V, Ravikumar D, Kesavan R, Venkatesan K, Saminathan R, Kannadasan R, Sudhakaran M, Alsharif MH, Geem ZW, Hong J. A Case Study on Renewable Energy Sources, Power Demand, and Policies in the States of South India—Development of a Thermoelectric Model. Sustainability . 2022; 14(14):8882. https://doi.org/10.3390/su14148882
Loganathan, Vijayaraja, Dhanasekar Ravikumar, Rupa Kesavan, Kanakasri Venkatesan, Raadha Saminathan, Raju Kannadasan, Mahalingam Sudhakaran, Mohammed H. Alsharif, Zong Woo Geem, and Junhee Hong. 2022. "A Case Study on Renewable Energy Sources, Power Demand, and Policies in the States of South India—Development of a Thermoelectric Model" Sustainability 14, no. 14: 8882. https://doi.org/10.3390/su14148882
Article Metrics
Article access statistics, further information, mdpi initiatives, follow mdpi.
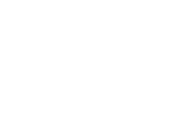
Subscribe to receive issue release notifications and newsletters from MDPI journals
- Resource Library
- FAQ Collections
- Upcoming Training
- Learning Pathways
- On-Demand Training
- Ask a Question
- Request In-Depth Assistance
- Find a Grantee
- Awards and Allocations
- Contact Information
- Reports & Plans
- Project Descriptions
- Email Updates
- Renew300: Advancing Renewable Energy in Affordable Housing
- Renewable Energy Resources
Renewable Energy Resources - Case Studies
The following case studies are examples of the successful introduction of renewables in federally-assisted properties. Click the case study title to view the full case study.
Denver Housing Authority: Leveraging Power Purchase Agreements for Scattered Site Solar Installations

Activity Type: Solar Photovoltaic
Project Details: Retrofit
Size/Rating: 2.5 mW; 10470 panels; Average 3.8kW per unit
On Site Generation: 3,397,576kWh/yr; Average: 5,101 kWh per unit/year
Cost: $10 million (Avg $4.00/ watt)
The Housing Authority of the City and County of Denver launched a public-private partnership to install solar photovoltaic systems across its portfolio of scattered-site, single family residential buildings. The installations are financed through a Power Purchase Agreement (PPA) with a solar provider that enables the PHA to achieve solar installations with no up-front capital costs. Under the PPA, the meter holders would pay for the power generated from the installed systems, initially priced at a rate roughly comparable to the current rates. Energy savings would occur in out-years as utility rates increased beyond the energy rate specified in the PPA.
EAH Housing: LIHTC Program Advances Whole Project Solar Systems without Added Subsidies in Richmond, CA

Project Details: Rehabilitation
Size/Rating: 900kW; 4,285 panels
On Site Generation: 1,220,832 kWh; 60-80% of community electric demands
Cost Savings: $154,000 per year
Cost: $ 8 million
At the time of construction, the 900 kW photovoltaic system at Crescent Park, an affordable housing complex in Richmond, California, was the largest solar system installed on a multifamily affordable housing project in the nation. The renewable investments at Crescent Park were undertaken in conjunction with the acquisition and rehabilitation of the property by EAH. This was an ideal time to incorporate renewable investments in the project’s capital plan because a variety of funding resources in play, and in many states preferences or added resources can be secured for energy investments. Through LIHTC, federal renewable investment tax credits and additional financing through the California Solar Initiative program, EAH was able to include an ambitious solar retrofit plans for the property.
El Paso Housing Authority: Renewables Provide Foundation for Zero Net Energy

Activity Type: Net Zero Project, Solar Photovoltaic, Wind Turbine | Project Details: New Construction
Size/Rating: 185kW; 165 kW PV; 710 panels; 20 kW Wind Turbines (2)
On Site Generation: Over 330,000 kWh/yr (Solar PV); Offset 4,190 kWh/unit/yr
Cost Savings: $43,245 ; $544 per unit | Cost: Solar: $966,000 | Total Project Costs: $10.9 million
Paisano Green Community is a newly constructed 73-unit senior housing facility located in central El Paso. Paisano Green Community is unique compared to other energy efficient buildings since it is part of a vanguard of new Zero Net Energy (ZNE) developments. Through innovative passive and active building systems, the project will minimize its energy consumption. To get to ZNE, renewable energy systems are used to offset the heating, cooling and plug loads demanded by occupants, such that the energy produced on site will equal or exceed energy consumed on site. This requires a deep a comprehensive understanding of climate conditions, building science, the performance of building systems, and occupant behavior.
Santa Barbara County Housing Authority: Public Housing Authority Portfolio Wide Solar Installation

Size/Rating: 1.7 mW; 7,200 panels
On Site Generation: 2.6 million kWh/yr; offsets 100% of tenant electric use
Cost Savings: $300,000 (@$0.15/kWh)
Cost: $12.25 million
The Housing Authority of the County of Santa Barbara (HACSB) has successfully implemented a portfolio-wide renewable energy strategy offsetting 100% of the electrical consumption at 21 properties and HACSB’s administration buildings. The 1.7 mW project involved the installation of over 1,700 solar photovoltaic panels on 250 buildings serving 863 dwelling units located in 6 permitting jurisdictions served by 3 different utility companies and is the largest renewable energy project undertaken by a public housing authority to date.
National Housing Trust/Enterprise: National Housing Trust Renewable Solar Financing Model

Implementation Model: Solar Financing Model
Organization Type: Affordable housing
Barrier: Financing solar energy in affordable multifamily housing
Solution: The creation of a separate business entity, NHT Renewable, to own, operate, and manage solar installations.
The National Housing Trust/Enterprise Preservation Corporation is an affiliate of the National Housing Trust, a non-profit engaged in preserving affordable housing. In order to combat the impact of volatile energy prices and harness more environmentally-friendly energy sources, NHT/Enterprise Preservation Corporation installed solar systems on its multifamily affordable housing properties and is working with other multifamily housing owners to do the same. The initiative established to own and operate the systems is called NHT Renewable. The first major NHT Renewable project was completed in Fall 2014—the installation of 14 solar systems across 13 buildings in Washington, D.C.

Better Buildings Challenge
This website provides information on the Better Buildings Challenge, an initiative that supports commercial and industrial building owners by providing technical assistance and proven solutions to energy efficiency. Website resources include best practices, guides, tools, and webinars related to the Better Buildings Challenge and its partners and allies.
More case studies can be found on the following websites:
HUD Office of Policy Development and Research
Bringing Solar Energy to Underserved Communities in Massachusetts
Green Building Information Gateway
Special Collection: Federally Assisted Multifamily Renewables
Special Collection: Multi-Family Projects with On-Site Renewables
- Phone This field is for validation purposes and should be left unchanged.
- Climate Change
- Policy & Economics
- Biodiversity
- Conservation
Get focused newsletters especially designed to be concise and easy to digest
- ESSENTIAL BRIEFING 3 times weekly
- TOP STORY ROUNDUP Once a week
- MONTHLY OVERVIEW Once a month
- Enter your email *
- Email This field is for validation purposes and should be left unchanged.
Case Study: Cost-Benefit Analysis of Renewable Energy Sources
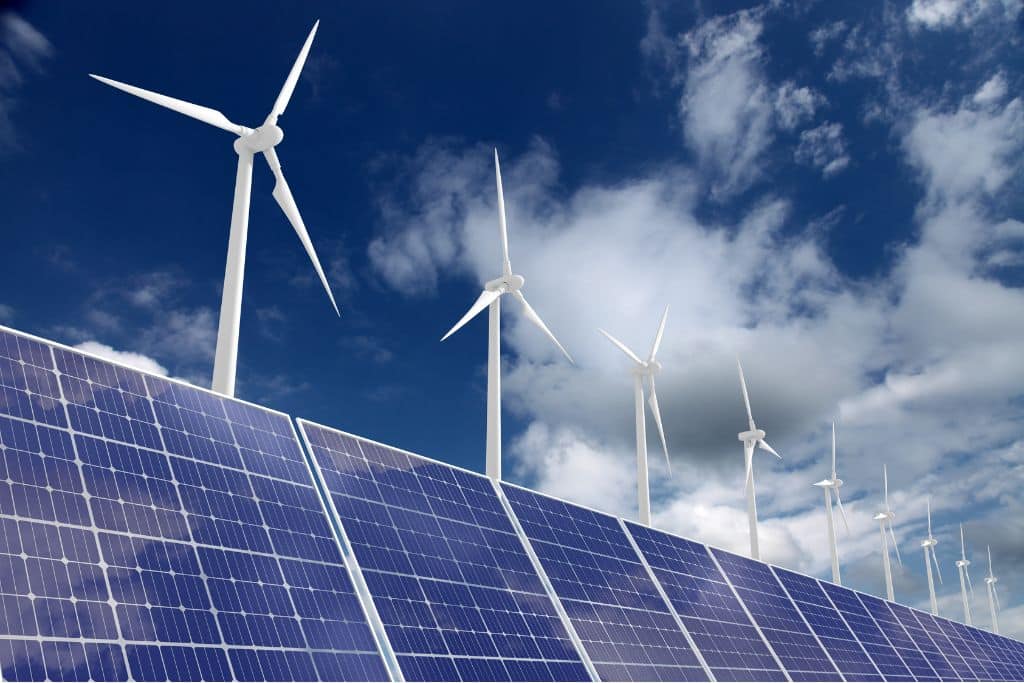
In this article, we explore the European Union’s commitment to renewable energy and the rise of ‘prosumer’ economics, as well as the efficient land use and environmental impact of anaerobic digestion plants (ABPs) for renewable energy production. We will also examine a case study in Montenegro, highlighting the effectiveness of renewable energy investments in reducing greenhouse gas emissions and the pressing need for cost-effective emission reduction strategies within the energy sector.
Renewable energy refers to environmentally friendly sources of energy that can be naturally replenished. They have the advantage of causing minimal harm to the environment while producing electricity. Wind , solar , hydroelectric , and geothermal sources are examples of renewable sources that generate electricity. They are not only cleaner but also cheaper and easier to produce than any fossil fuel.
Although there are expenses associated with harnessing these resources, their affordability enables scientists and engineers to exploit them effectively and facilitate the green transition.
EU’s Drive Towards Renewable Energy and ‘Prosumer’ Economics
The 27-member bloc conducted financial evaluations of green projects to determine their feasibility. It looked at things like cash flow (how money moves in and out), payback time (when you make back your initial investment), net present value (what future money is worth today), and internal rate of return (how profitable the investment is).
The analysis , published in 2021, found that if regular electricity users start generating their own power using renewable sources, they can become so-called “prosumers”: individuals who both consume and produce their own electricity using renewable sources. This approach can contribute to energy savings by reducing the distance electricity must travel from power plants to homes. The profitability of becoming a prosumer depends on factors such as setup costs, the amount of power consumed at home, and the surplus power sold back to the grid.
ABP Land Efficiency and Environmental Impact Assessment
A decade ago, a team of researchers at the University of Montenegro collaborated with the Ministry of Transport and Maritime Affairs to create a mode to evaluate and enhance the land requirements of anaerobic digestion plants (ABPs) while simultaneously minimising their environmental footprint. This comprehensive model was tailored to account for the distinct land requirements linked to ABPs, ensuring efficient land utilisation in the process.
To gauge the efficiency of land use in ABPs, these were compared to other energy sources like photovoltaic panels, onshore wind systems, and thermal power stations. The analysis revealed that ABPs are relatively efficient in terms of land utilisation for energy production.
Beyond land use, the evaluation of ABPs extends to their broader environmental footprint. This assessment encompasses factors such as greenhouse gas emissions, consumption of non-renewable resources, and the potential impacts of ABPs on local communities and ecosystems.
Matching the right renewable energy system to your energy needs is essential for both cost savings and environmental benefits and ensuring this alignment is crucial to maximise the associated benefits of using renewable energy sources.
You might also like: Renewables Will Dominate World’s Electricity Demand Through 2025, IEA Report Says
Assessing GHG Reduction in Montenegro: Analysis and Limitations
The aforementioned 2021 analysis includes a case study in Montenegro which focuses on the country’s significant dependence on imported liquid and gaseous fossil fuels. This reliance is closely linked to Montenegro’s energy sector, which heavily utilises imported fossil fuels for energy production, resulting in substantial greenhouse gas emissions (GHGs), a primary contributor to global warming.

Montenegro has significantly bolstered its investments in renewable energy sources from 2016 to 2021, resulting in a remarkable 20% reduction in GHG emissions. These notable achievements are particularly prominent within the energy sector. Currently, renewable energy sources contribute to around 35% of Montenegro’s overall energy production. Moreover, the nation has strategically crafted and implemented various initiatives aimed at elevating energy efficiency and curbing emissions, reinforcing its commitment to a sustainable and eco-friendly future.
To assess the economic feasibility of GHG reduction measures, the scientists behind the study conducted an economic analysis. Using a dataset spanning a decade, they calculated the present value of monetary units. Key economic indicators, such as net present value and benefit-cost ratio, were employed to evaluate the cost-effectiveness of various measures.
The analysis underscores the substantial positive impact of implementing economic and structural changes within the metal industry. These changes likely encompass improvements in energy efficiency, emissions reductions from industrial processes, and a potential transition to cleaner energy sources within the metal industry. As a result, emissions associated with this sector have significantly decreased, making a substantial contribution to the overall reduction in greenhouse gas emissions. However, the study also emphasises a crucial point: there is a pressing need for further greenhouse gas emissions reductions, particularly within the energy sector. This indicates that while progress has been made in the metal industry, there is still a need for additional emissions reductions at a broader scale, which may encompass the entire country or even global efforts.
This might involve a shift towards cleaner and more sustainable energy sources, enhanced energy efficiency practices, and the implementation of policies promoting renewable energy generation.
The study does have limitations, notably regarding the accuracy of investment cost estimates for certain measures. Future research should include cluster analysis to group measures based on their emissions impact. Additionally, addressing data limitations and conducting more precise analyses in specific areas of the research is essential.
Renewable energy resources offer an environmentally friendly and cost-effective solution for cleaner electricity generation. The efficient land use in (ABPs) for renewable energy production is a promising aspect, as ABPs demonstrate relatively low land requirements compared to other energy sources. However, it is crucial to assess their broader environmental impact, including greenhouse gas emissions and community effects.
The case study in Montenegro highlights the effectiveness of renewable energy investments in reducing emissions, emphasising the need for cost-effective emission reduction strategies in the energy sector. Despite study limitations, these findings underscore the importance of advancing cleaner and sustainable energy systems globally, balancing power needs with environmental preservation.
You might also like: 7 Interesting Renewable Energy Facts
About the Author
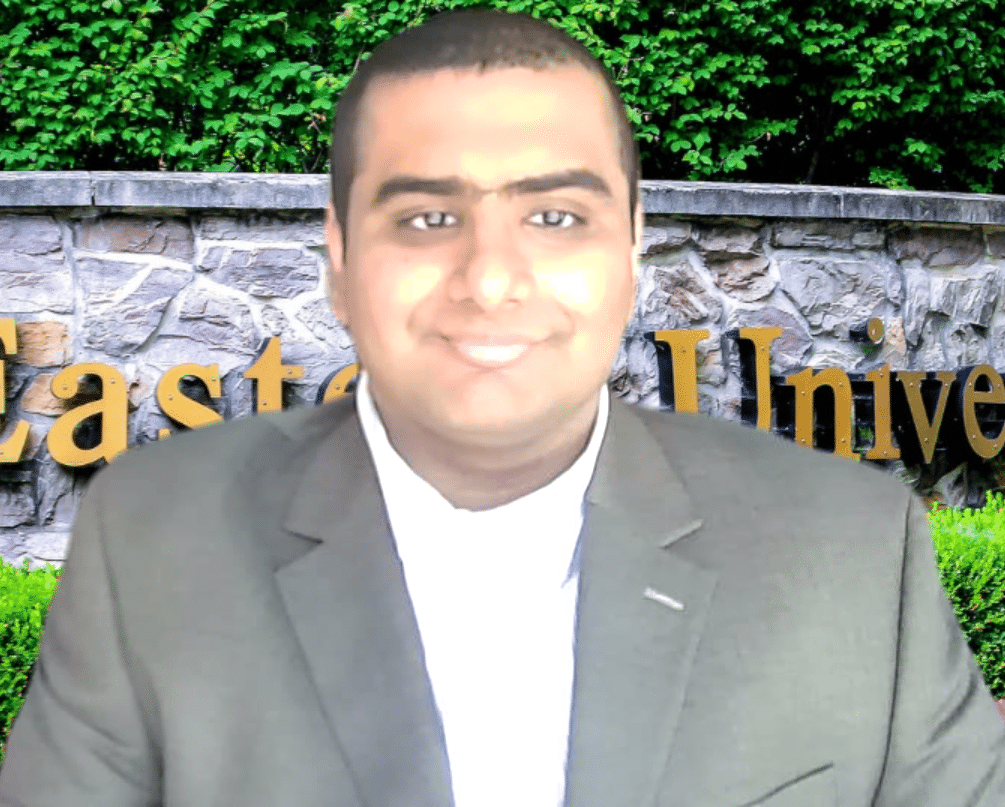
Karnik Aswani
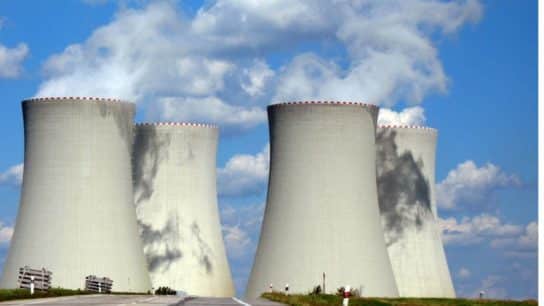
The Advantages and Disadvantages of Nuclear Energy
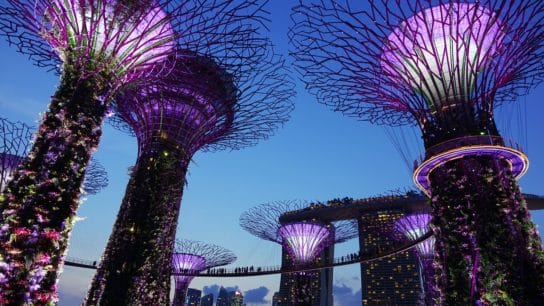
Top 7 Smart Cities in the World in 2024
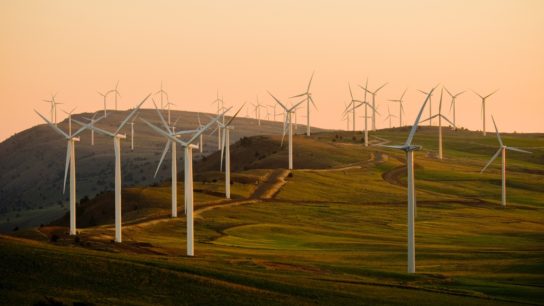
What the Future of Renewable Energy Looks Like
Hand-picked stories weekly or monthly. We promise, no spam!
- Comments This field is for validation purposes and should be left unchanged.
Boost this article By donating us $100, $50 or subscribe to Boosting $10/month – we can get this article and others in front of tens of thousands of specially targeted readers. This targeted Boosting – helps us to reach wider audiences – aiming to convince the unconvinced, to inform the uninformed, to enlighten the dogmatic.
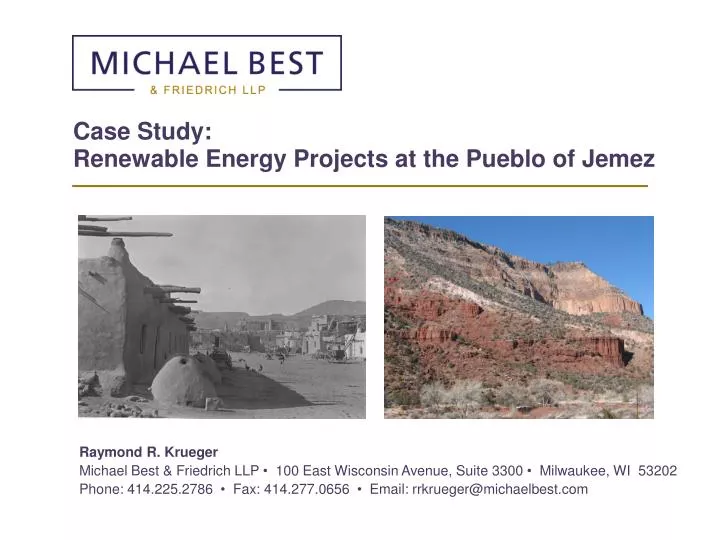
Case Study: Renewable Energy Projects at the Pueblo of Jemez
Mar 16, 2019
200 likes | 320 Views
Case Study: Renewable Energy Projects at the Pueblo of Jemez. Raymond R. Krueger Michael Best & Friedrich LLP • 100 East Wisconsin Avenue, Suite 3300 • Milwaukee, WI 53202 Phone: 414.225.2786 • Fax: 414.277.0656 • Email: [email protected]. The Pueblo of Jemez.
Share Presentation
- project benefits
- rrkrueger michaelbest
- service life
- project benefits cont
- state production tax credits
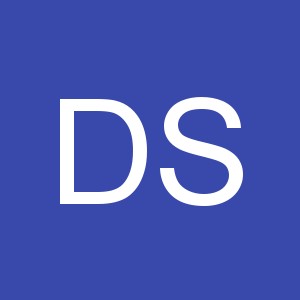
Presentation Transcript
Case Study:Renewable Energy Projects at the Pueblo of Jemez Raymond R. Krueger Michael Best & Friedrich LLP • 100 East Wisconsin Avenue, Suite 3300 • Milwaukee, WI 53202 Phone: 414.225.2786 • Fax: 414.277.0656 • Email: [email protected]
The Pueblo of Jemez • Federally-recognized Tribe • 45 Miles NW of Albuquerque, NM • 2,500 Tribal members in village of Walatowa; 3,000 Tribal members total • Only Towa-speaking Tribe, very traditional • Has occupied the Jemez Valley for over 800 years
Summary of the Jemez 3.5 MW ac Solar Project • Generate 3.5 MW ac of solar power using proven single axis flat plate PV on 30 acres of tribal trust land • Interconnect with Jemez Mountains Electrical Cooperative system at existing 69kV transmission line at site • Sell the power and Renewable Energy Credits generated by the project to a local utility • Revenue from power/REC sales go to Pueblo for much needed infrastructure improvements and community services
Summary of the Jemez 3.5 MW ac Solar Project (cont’d) • Remains efficient on cloudy days • Lighter weight means better choice for mixed soil conditions • 30-year service life • Single-Axis, Flat Plate Solar Arrays • 30-year track record, highly reliable, easily maintained
Summary of the Jemez 3.5 MW ac Solar Project (cont’d) ConceptualSite Plan – 30 Acres
Summary of the Jemez 3.5 MW ac Solar Project (cont’d) Looking northeast from atop the mesita across main portion of solar site.
Project Benefits • Environmental Benefits: • A coal-fired power plant emits 2,249 lbs. of CO2 gas per MW hour • Over the service life of the equipment, the Jemez Solar Project will offset over 278,876 tons of CO2 • First commercial scale, grid-tied solar project on tribal land nationwide • Project could be replicated by other tribes
Project Benefits (cont’d) • Fiscal/Social Benefits: • Provide sustainable, profitable revenue stream to Pueblo • Could improve major infrastructure problems at the Pueblo • Creates track record for Pueblo as a competent renewable energy project developer leading to additional solar, wind, geothermal, and biomass projects • Sets positive example for Jemez students pursuing college and careers in hopes of contributing to the Pueblo and their people Source: U.S. EPA: The Emissions & Generation Resource Integrated Database (eGRID), 2008. http://www.epa.gov/solar/energy-resources/egrid/faq.html
How is Project Being Funded? • The Pueblo does not have $20 million it will take to build the project and taking out a loan that big does not work economically • Tax credits work the same as bringing money to the table. They are the same as cash to the bank (equity) • Our Finance Model, developed by Red Mountain Energy Partners, uses three federal tax credits, the Investment Tax Credit, the New Market Tax Credit, and State Production Tax Credits • Combined, these three tax credits cover approximately half of the project cost • The remaining half will be funded by a loan
What’s Next? • Complete negotiations on Power Purchase Agreement • Form a Project LLC with finance partners • Complete a geotechnical study and grading and drainage plan • Procure construction services • Break ground Fall, 2010 • Continue development of our geothermal and biomass projects
Questions? Raymond R. Krueger Michael Best & Friedrich LLP 100 East Wisconsin Avenue Suite 3300 Milwaukee, WI 53202 414.225.2786 [email protected]
- More by User
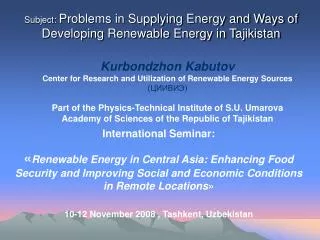
MAN AND ENERGY A case for Sustainable Living through Renewable and Green Energy
MAN AND ENERGY A case for Sustainable Living through Renewable and Green Energy. Ali Keyhani Professor of Electrical and Computer Engineering The Ohio State University Columbus, OH-43210 k [email protected]. ABSTRACT.
1.21k views • 84 slides
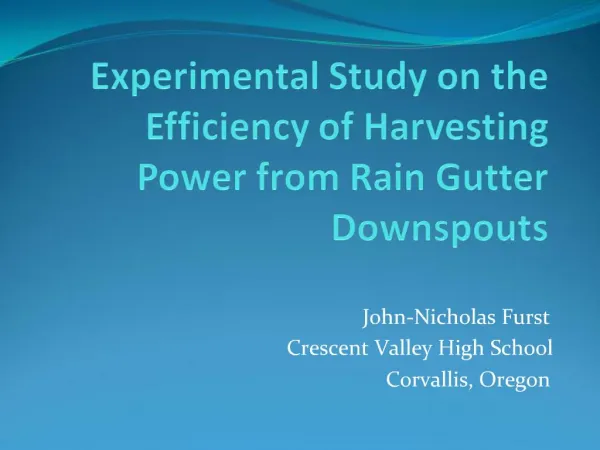
Experimental Study on the Efficiency of Harvesting Power from Rain Gutter Downspouts
Goal. Determine how much energy can be produced from rain water running down gutter downspouts.. Renewable Energy and Hydroelectricity. Produced by harnessing the energy of moving water and converting it into electricity.Supplies 715,000 megawatts of electricity or 19% of the world's electricity. 63% of the total energy harvested from renewable sources. .
1.39k views • 13 slides
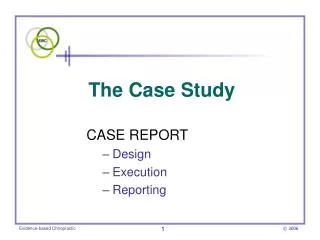
The Case Study
CASE REPORT Design Execution Reporting. The Case Study. The case may be an individual, an event, a policy, etc. e.g., a case of deafness and SMT (Harvey Lillard). Types of case studies. Case reports in the larger scheme of things:. Randomized clinical trial Cohort study
1.64k views • 65 slides
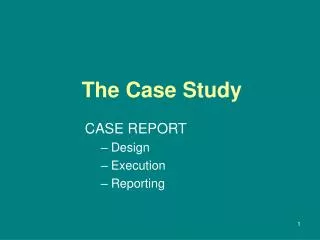
1.37k views • 66 slides
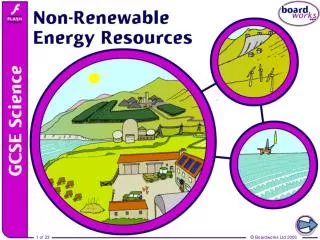
What do renewable and non-renewable mean?
What do renewable and non-renewable mean?. Energy resources can be classified into two groups. Renewable. Non-renewable. Renewable energy resources can be replaced or regenerated and will never run out (at least not for a very long time).
1.55k views • 33 slides
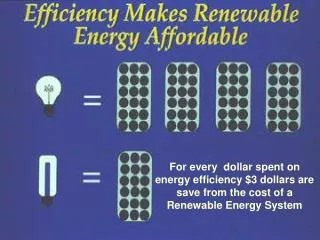
For every dollar spent on energy efficiency $3 dollars are save from the cost of a Renewable Energy System
For every dollar spent on energy efficiency $3 dollars are save from the cost of a Renewable Energy System. Energy is transported from the Sun to Earth via the Infrared Spectrum and via Photons . A Square of
1.1k views • 90 slides
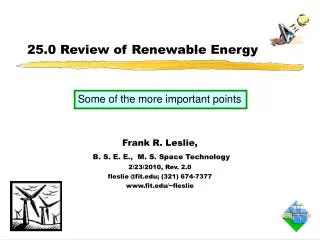
25.0 Review of Renewable Energy
25.0 Review of Renewable Energy. Some of the more important points. Frank R. Leslie, B. S. E. E., M. S. Space Technology 2/23/2010, Rev. 2.0 fleslie @fit.edu; (321) 674-7377 www.fit.edu/~fleslie. In Other News . . . . Crude oil continues at ~$50/bbl
1.09k views • 79 slides
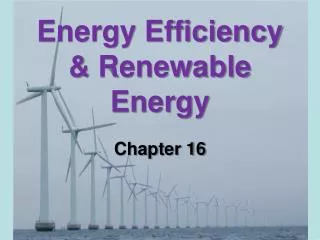
Energy Efficiency & Renewable Energy
Energy Efficiency & Renewable Energy. Chapter 16. Core Case Study: Iceland’s Vision of a Renewable-Energy Economy (1). Supplies 75% of its primary energy and almost all of its electrical energy using Geothermal energy Hydroelectric power No fossil fuel deposits: imports oil
1.44k views • 84 slides
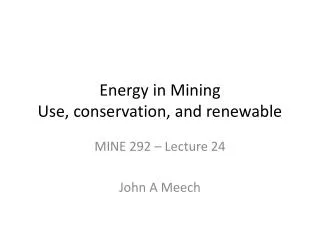
Energy in Mining Use, conservation, and renewable
Energy in Mining Use, conservation, and renewable. MINE 292 – Lecture 24 John A Meech. Outline. Energy Use and Conservation Grinding Ventilation Fuel for Transportation Heat Sources of Power Grid power (large-scale hydropower in B.C.) Run-of-River hydropower Geothermal Solar and Wind
1.2k views • 98 slides
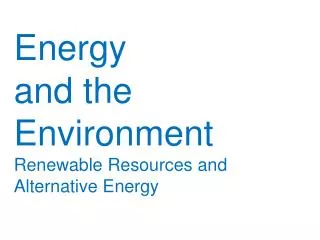
Energy and the Environment Renewable Resources and Alternative Energy
Energy and the Environment Renewable Resources and Alternative Energy. Energy efficiency calculation: energy efficiency (in %) = energy in/energy out X 100
881 views • 73 slides
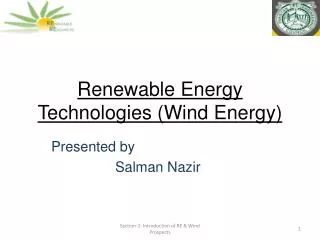
Renewable Energy Technologies (Wind Energy)
Renewable Energy Technologies (Wind Energy). Presented by Salman Nazir. Presentation Highlights. Introduction to Renewable Resources Pvt Limited Need to Introduce Renewable Energies in Power sector (Introduction of RE) Global Wind Market Potential to work in wind Sector-Pakistan
1.33k views • 86 slides
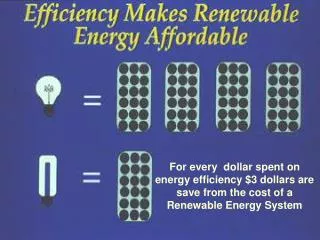
1.02k views • 90 slides
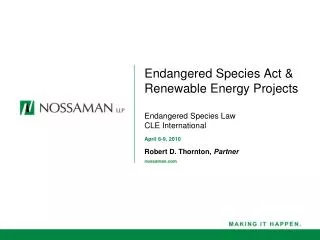
Endangered Species Act & Renewable Energy Projects
Endangered Species Act & Renewable Energy Projects. Endangered Species Law CLE International. April 8-9, 2010. Robert D. Thornton, Partner. The Endangered Species Act Meets Climate Change.
843 views • 55 slides
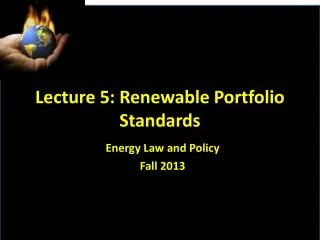
Lecture 5: Renewable Portfolio Standards
Lecture 5: Renewable Portfolio Standards. Energy Law and Policy Fall 2013. Class Schedule. 8/26: INTRODUCTION AND BACKGROUND 8/28: Forces controlling energy policy; Jim Halloran, PNC Bank. 9/4: Energy Policy Overview and History of Energy Regulation 9/9: History of Regulation
1.02k views • 79 slides
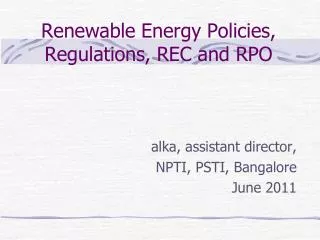
Renewable Energy Policies, Regulations, REC and RPO
Renewable Energy Policies, Regulations, REC and RPO. alka, assistant director, NPTI, PSTI, Bangalore June 2011. ENERGY. Then ……… & Now …. Energy use has changed a great deal since people relied solely on the sun, their own strong bodies or beasts of burden as energy resources.
1.14k views • 75 slides
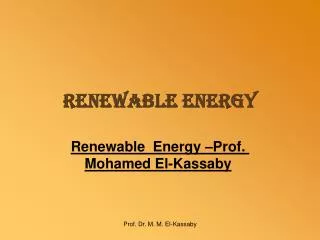
Renewable Energy
Renewable Energy. Renewable Energy –Prof. Mohamed El-Kassaby. The energy production in Egypt through the year 2005 was 100996 GWh. 73.9% of the total production was produced from thermal power station.
1.11k views • 88 slides
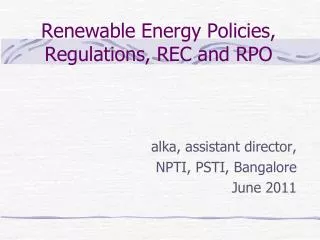
987 views • 75 slides
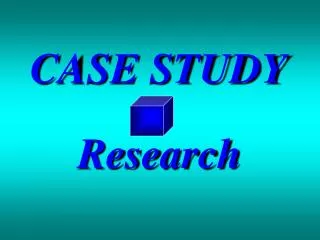
CASE STUDY. Research. Case Study Defined. Yin (1994). Merriam (1988). A qualitative case study is an intensive, holistic description and analysis of a single instance, phenonomenon, or social unit (p. 21).
2.03k views • 52 slides
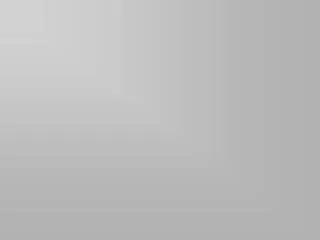
ENERGIES FOR A SUSTAINABLE FUTURE
ENERGIES FOR A SUSTAINABLE FUTURE. The potential of Renewable Energies. F.P. Neirac – CEP – Ecole des Mines. ENERGIES FOR A SUSTAINABLE FUTURE. History Energy and economy The case of Renewable Energies. Energy. Classification PV Solar concentrating technologies Wind Geothermy
1.4k views • 112 slides
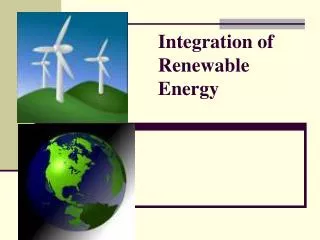
Integration of Renewable Energy
Integration of Renewable Energy. Outline of this presentation. Introduction Basics of Renewable Technologies Scenario of Renewable Energy generation in India Issues involved in Grid Integration of RE: International Experience The Road ahead.
1.43k views • 93 slides
The Understand Energy Learning Hub is a cross-campus effort of the Precourt Institute for Energy .

Introduction to Renewable Energy
Exploring our content.
Fast Facts View our summary of key facts and information. ( Printable PDF, 270 KB )
Before You Watch Our Lecture Maximize your learning experience by reviewing these carefully curated readings we assign to our students.
Our Lecture Watch the Stanford course lecture.
Additional Resources Find out where to explore beyond our site.

Fast Facts About Renewable Energy
Principle Energy Uses: Electricity, Heat Forms of Energy: Kinetic, Thermal, Radiant, Chemical
The term “renewable” encompasses a wide diversity of energy resources with varying economics, technologies, end uses, scales, environmental impacts, availability, and depletability. For example, fully “renewable” resources are not depleted by human use, whereas “semi-renewable” resources must be properly managed to ensure long-term availability. The most renewable type of energy is energy efficiency, which reduces overall consumption while providing the same energy service. Most renewable energy resources have significantly lower environmental and climate impacts than their fossil fuel counterparts.
The data in these Fast Facts do not reflect two important renewable energy resources: traditional biomass, which is widespread but difficult to measure; and energy efficiency, a critical strategy for reducing energy consumption while maintaining the same energy services and quality of life. See the Biomass and Energy Efficiency pages to learn more.
Significance
14% of world 🌎 9% of US 🇺🇸
Electricity Generation
30% of world 🌎 21% of US 🇺🇸
Global Renewable Energy Uses
Electricity 65% Heat 26% Transportation 9%
Global Consumption of Renewable Electricity Change
Increase: ⬆ 33% (2017 to 2022)
Energy Efficiency
Energy efficiency measures such as LED light bulbs reduce the need for energy in the first place
Renewable Resources
Wind Solar Ocean
Semi-Renewable Resources
Hydro Geothermal Biomass

Renewable Energy Has Vast Potential to Meet Global Energy Demand
Solar >1,000x global demand Wind ~3x global demand
Share of Global Energy Demand Met by Renewable Resources
Hydropower 7% Wind 3% Solar 2% Biomass <2%
Share of Global Electricity Generation Met by Renewable Resources
Hydropower 15% Wind 7% Solar 5% Biomass & Geothermal <3%
Global Growth
Hydropower generation increase ⬆6% Wind generation increase ⬆84% Solar generation increase ⬆197% Biofuels consumption increase ⬆23% (2017-2022)
Largest Renewable Energy Producers
China 34% 🇨🇳 US 10% 🇺🇸 of global renewable energy
Highest Penetration of Renewable Energy
Norway 72% 🇳🇴 of the country’s primary energy is renewable
(China is at 16%, the US is at 11%)
Largest Renewable Electricity Producers
China 31% 🇨🇳 US 11% 🇺🇸 of global renewable electricity
Highest Penetration of Renewable Electricity
Albania, Bhutan, CAR, Lesotho, Nepal, & Iceland 100%
Iceland, Ethiopia, Paraguay, DRC, Norway, Costa Rica, Uganda, Namibia, Eswatini, Zambia, Tajikistan, & Sierra Leone > 90% of the country’s primary electricity is renewable
(China is at 31%, the US is at 22%)
Share of US Energy Demand Met by Renewable Resources
Biomass 5% Wind 2% Hydro 1% Solar 1%
Share of US Electricity Generation Met by Renewable Resources
Wind 10% Hydropower 6% Solar 3% Biomass 1%
US States That Produce the Most Renewable Electricity
Texas 21% California 11% of US renewable energy production
US States With Highest Penetration of Renewable Electricity
Vermont >99% South Dakota 84% Washington 76% Idaho 75% of state’s total generation comes from renewable fuels
Renewable Energy Expansion Policies
The Inflation Reduction Act continued tax credits for new renewable energy projects in the US.
Production Tax Credit (PTC)
Tax credit of $0.0275/kWh of electricity produced at qualifying renewable power generation sites
Investment Tax Credit (ITC)
Tax credit of 30% of the cost of a new qualifying renewable power generation site
To read more about the credit qualifications, visit this EPA site .
Resource (Renewables) | Unsubsidized LCOE* | LCOE with ITC/PTC Tax Subsidy |
---|---|---|
Wind (Onshore) | $24 - $75 | $0 - $66 (PTC) |
Solar PV (Utility Scale) | $24 - $96 | $16 - $80 (ITC) $0 - $77 (PTC) |
Solar + Storage (Utility Scale) | $46 - $102 | $31 - $88 (ITC) |
Geothermal | $61 - $102 | $37 - $87 |
Wind (Offshore) | $72 - $140 | $56 - $114 (PTC) |
Solar PV (Rooftop Residential) | $177 - $282 | $74 - $229 (ITC) |
Wind + Storage (Onshore) | $24 - $75 | $0 - $66 (PTC) |
Resource (Non-Renewables) | Unsubsidized LCOE* |
---|---|
Natural Gas (combined cycle) | $39 - $101 |
Natural Gas Peaker Plants | $115 - $221 |
Coal | $68 - $166 |
Nuclear | $141 - $221 |
*LCOE (levelized cost of electricity) - price for which a unit of electricity must be sold for system to break even
Important Factors for Renewable Site Selection
- Resource availability
- Environmental constraints and sensitivities, including cultural and archeological sites
- Transmission infrastructure
- Power plant retirements
- Transmission congestion and prices
- Electricity markets
- Load growth driven by population and industry
- Policy support
- Land rights and permitting
- Competitive and declining costs of wind, solar, and energy storage
- Lower environmental and climate impacts (social costs) than fossil fuels
- Expansion of competitive wholesale electricity markets
- Governmental clean energy and climate targets and policies
- Corporate clean energy targets and procurement of renewable energy
- No fuel cost or fuel price volatility
- Retirements of old and/or expensive coal and nuclear power plants
- Most renewable resources are abundant, undepletable
- Permitting hurdles and NIMBY/BANANA* concerns
- Competition from subsidized fossil fuels and a lack of price for their social cost (e.g., price on carbon)
- Site-specific resources means greater need to transport energy/electricity to demand
- High initial capital expenditure requirements required to access fuel cost/operating savings
- Intermittent resources
- Inconsistent governmental incentives and subsidies
- Managing environmental impacts to the extent that they exist
*NIMBY - not in my backyard; BANANA - build absolutely nothing anywhere near anything
Climate Impact: Low to High

- Solar, wind, geothermal, and ocean have low climate impacts with near-zero emissions; hydro and biomass can have medium to high climate impact
- Hydro: Some locations have greenhouse gas emissions due to decomposing flooded vegetation
- Biomass: Some crops require significant energy inputs, land use change can release carbon dioxide and methane
Environmental Impact: Low to High
- Most renewable energy resources have low environmental impacts, particularly relative to fossil fuels; some, like biomass, can have more significant impacts
- No air pollution with the exception of biomass from certain feedstocks
- Can have land and habitat disruption for biomass production, solar, and hydro
- Potential wildlife impacts from wind turbines (birds and bats)
- Modest environmental impacts during manufacturing, transportation, and end of life
Updated January 2024
Before You Watch Our Lecture on Introduction to Renewable Energy
We assign videos and readings to our Stanford students as pre-work for each lecture to help contextualize the lecture content. We strongly encourage you to review the Essential reading below before watching our lecture on Introduction to Renewable Energy . Include the Optional and Useful readings based on your interests and available time.
- The Sustainable Energy in America 2024 Factbook (Executive Summary pp. 5-10) . Bloomberg New Energy Finance. 2024. (6 pages) Provides valuable year-over-year data and insights on the American energy transformation.
Optional and Useful
- Renewables 2024 Global Status Report (Global Overview pp. 10-39) . REN21. 2024. (30 pages) Documents the progress made in the renewable energy sector and highlights the opportunities afforded by a renewable-based economy and society.
Our Lecture on Introduction to Renewable Energy
This is our Stanford University Understand Energy course lecture that introduces renewable energy. We strongly encourage you to watch the full lecture to gain foundational knowledge about renewable energy and important context for learning more about specific renewable energy resources. For a complete learning experience, we also encourage you to review the Essential reading we assign to our students before watching the lecture.

Presented by: Kirsten Stasio , Adjunct Lecturer, Civil and Environmental Engineering, Stanford University; CEO, Nevada Clean Energy Fund (NCEF) Recorded on: May 15, 2024 Duration: 68 minutes
Table of Contents
(Clicking on a timestamp will take you to YouTube.) 00:00 Introduction 02:06 What Does “Renewable” Mean? 15:29 What Role Do Renewables Play in Our Energy Use? 27:12 What Factors Affect Renewable Energy Project Development?
Lecture slides available upon request .
Additional Resources About Renewable Energy
Stanford university.
- Precourt Institute for Energy Renewable Energy , Energy Efficiency
- Stanford Energy Club
- Energy Modeling Forum
- Sustainable Stanford
- Sustainable Finance Initiative
- Mark Jacobson - Renewable energy
- Michael Lepech - Life-cycle analysis
- Leonard Ortolano - Environmental and water resource planning
- Chris Field - Climate change, land use, bioenergy, solar energy
- David Lobell - Climate change, agriculture, biofuels, land use
- Sally Benson - Climate change, energy, carbon capture and storage
Government and International Organizations
- International Energy Agency (IEA) Renewables Renewables 2022 Report .
- National Renewable Energy Laboratory (NREL)
- US Department of Energy (DOE) Office of Energy Efficiency & Renewable Energy (EERE)
- US Energy Information Administration (EIA) Renewable Energy Explained
- US Energy Information Administration (EIA) Energy Kids Renewable Energy
- US Energy Information Administration (EIA) Today in Energy Renewables
Other Organizations and Resources
- REN21: Renewable Energy Policy Network for the 21st Century
- REN21 Renewables 2023 Global Status Report Renewables in Energy Supply
- BloombergNEF (BNEF)
- Carnegie Institution for Science Biosphere Sciences and Engineering
- The Solutions Project
- Renewable Energy World
- World of Renewables
- Energy Upgrade California
Next Topic: Energy Efficiency Other Energy Topics to Explore
Fast Facts Sources
- Energy Mix (World 2022): Energy Institute. Statistical Review of World Energy . 2023.
- Energy Mix (US 2022): US Energy Information Agency (EIA). Total Energy: Energy Overview, Table 1.3 .
- Electricity Mix (World 2022): Energy Institute. Statistical Review of World Energy . 2023.
- Electricity Mix (US 2022): US Energy Information Agency (EIA). Total Energy: Electricity, Table 7.2a.
- Global Solar Use (2022): REN21. Renewables 2023 Global Status Report: Renewables in Energy Supply , page 42. 2023
- Global Consumption of Renewable Electricity Change (2017-2022): Energy Institute. Statistical Review of World Energy . 2023.
- Renewable Energy Potential: Perez & Perez. A Fundamental Look at Energy Reserves for the Planet . 2009
- Share of Global Energy Demand (2022): Energy Institute. Statistical Review of World Energy . 2023.
- Share of Global Electricity Demand (2022): Energy Institute. Statistical Review of World Energy . 2023.
- Global Growth (2017-2022): Energy Institute. Statistical Review of World Energy . 2023.
- Largest Renewable Energy Producers (World 2022): International Renewable Energy Agency (IRENA). Renewable Capacity Statistics 2023 . 2023.
- Highest Penetration Renewable Energy (World 2022): Our World in Data. Renewable Energy . 2023.
- Largest Renewable Electricity Producers (World 2022): Energy Institute. Statistical Review of World Energy . 2023.
- Highest Penetration Renewable Electricity (World 2022): Our World in Data. Renewable Energy . 2023.
- Share of US Energy Demand (2022): Energy Information Administration (EIA). Electric Power Monthly. 2023.
- Share of Electricity Generation (2022): Energy Information Administration (EIA). Electric Power Monthly. 2023.
- States with Highest Generation (2022): Energy Information Administration (EIA). Electric Power Monthly. 2023.
- States with Highest Penetration (2021): Energy Information Administration (EIA). State Profile and Energy Estimates. 2023.
- LCOE of US Renewable Resources: Lazard. LCOE. April 2023.
- LCOE of US Non Renewable Resources: Lazard. LCOE. April 2023.
More details available on request . Back to Fast Facts
Got any suggestions?
We want to hear from you! Send us a message and help improve Slidesgo
Top searches
Trending searches
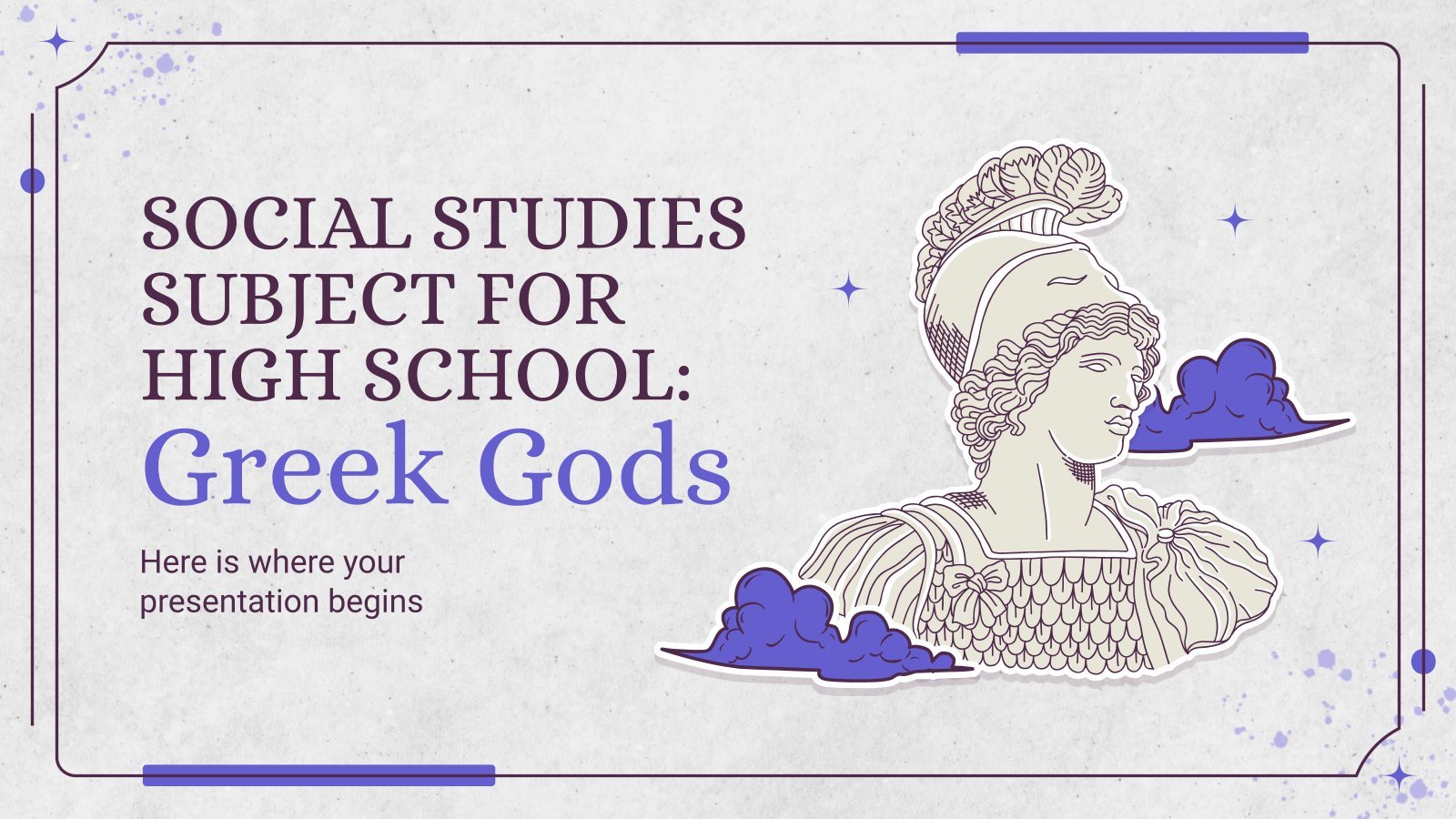
17 templates
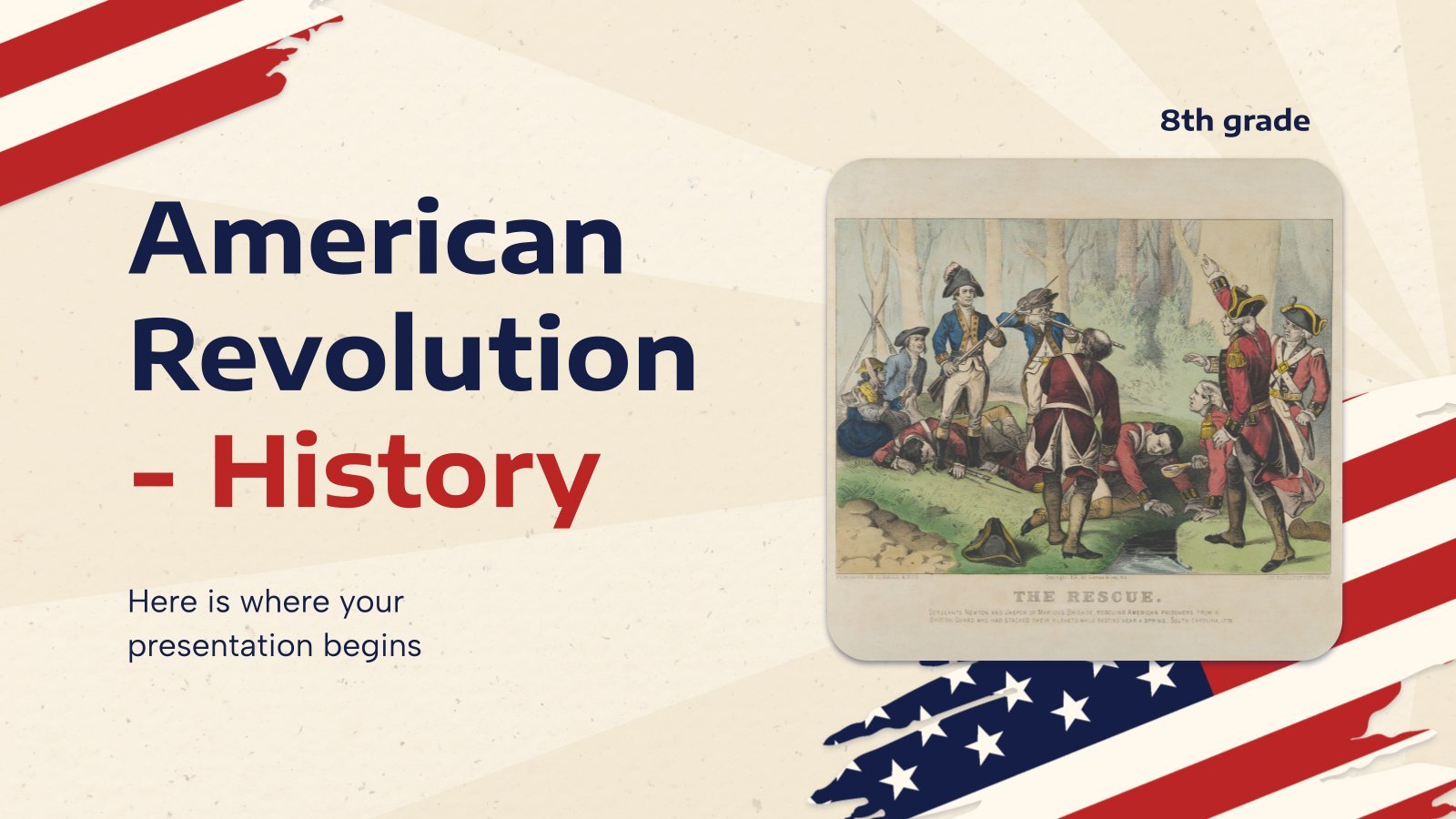
american history
85 templates
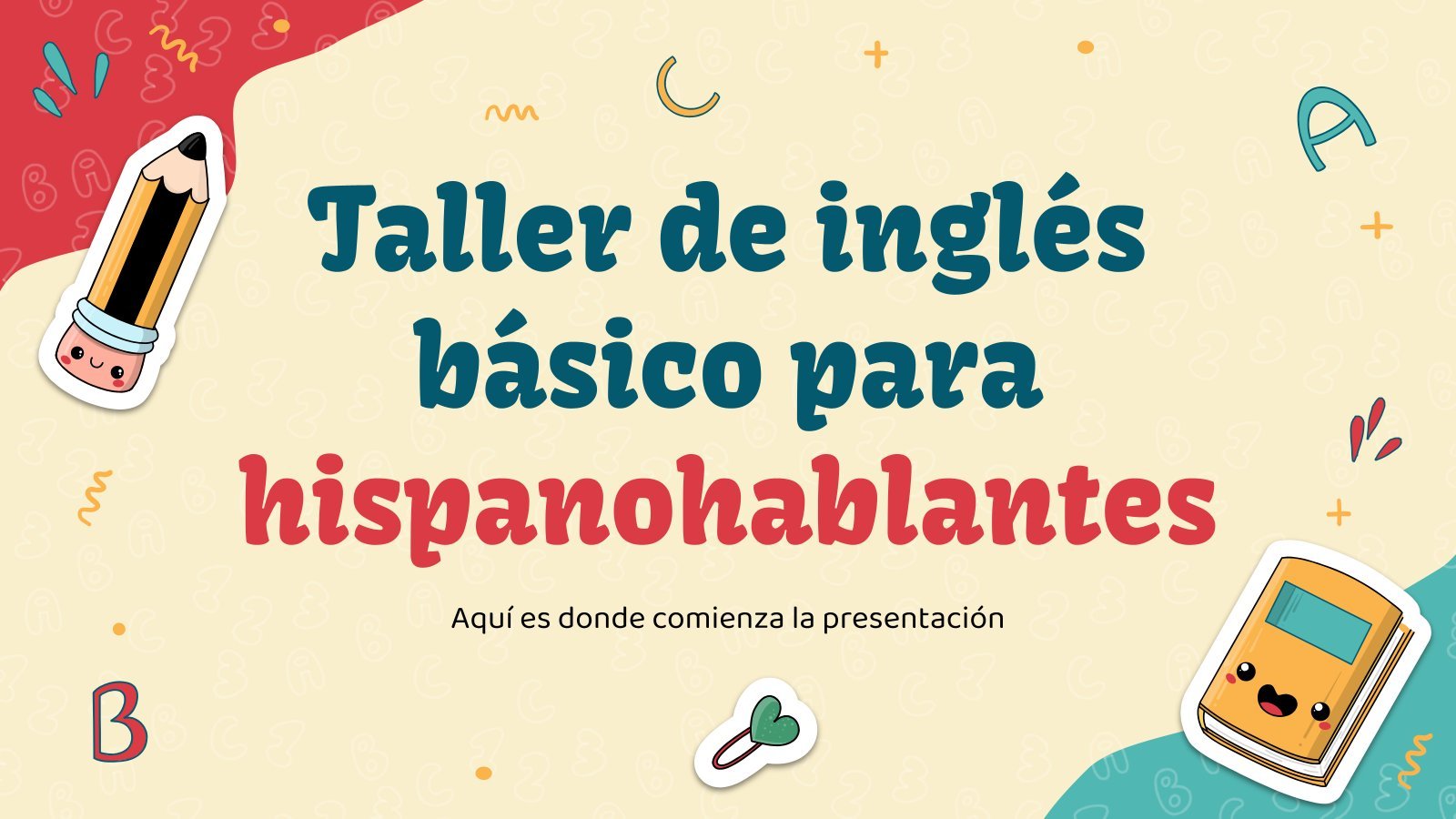
49 templates
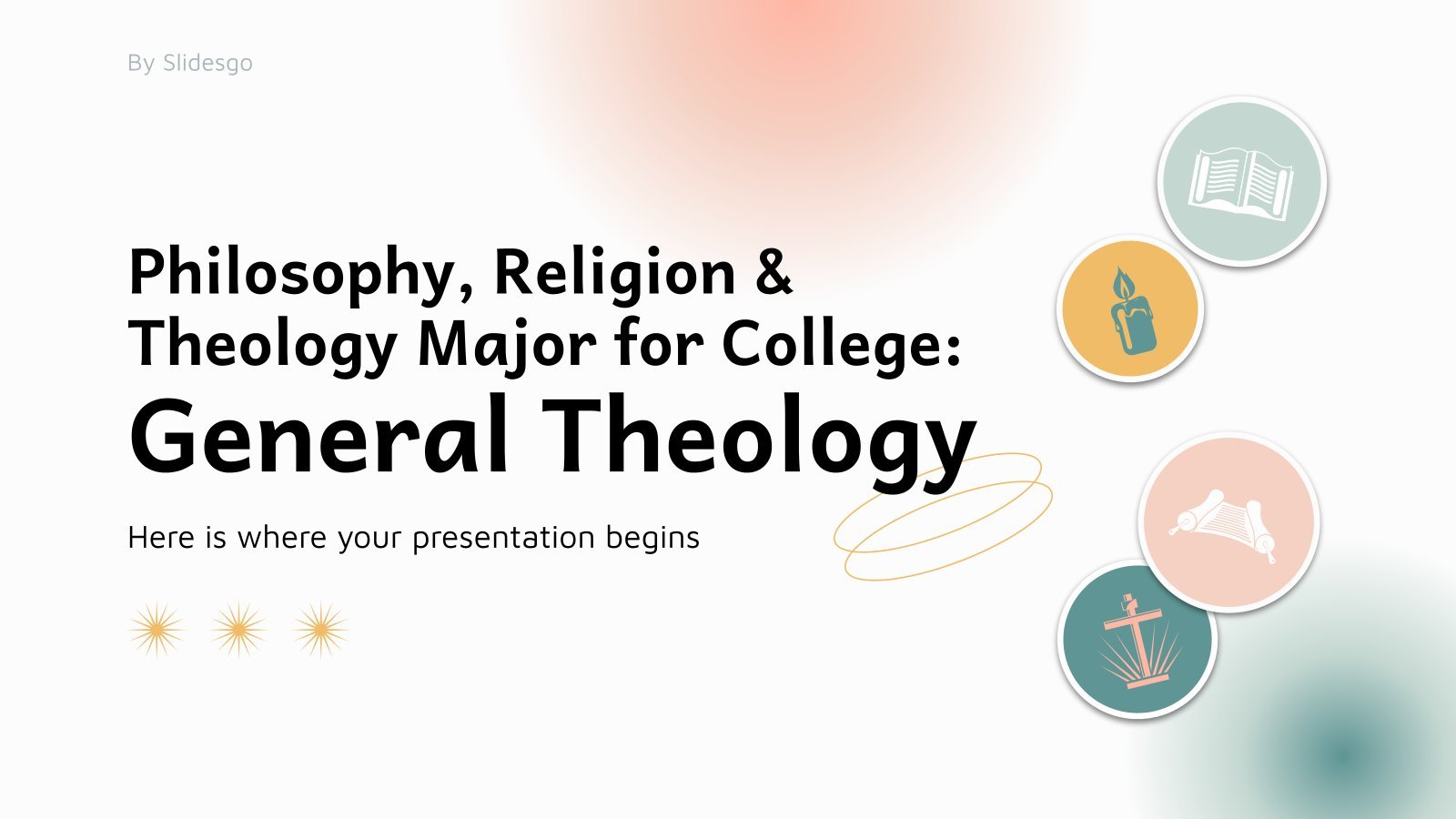
43 templates
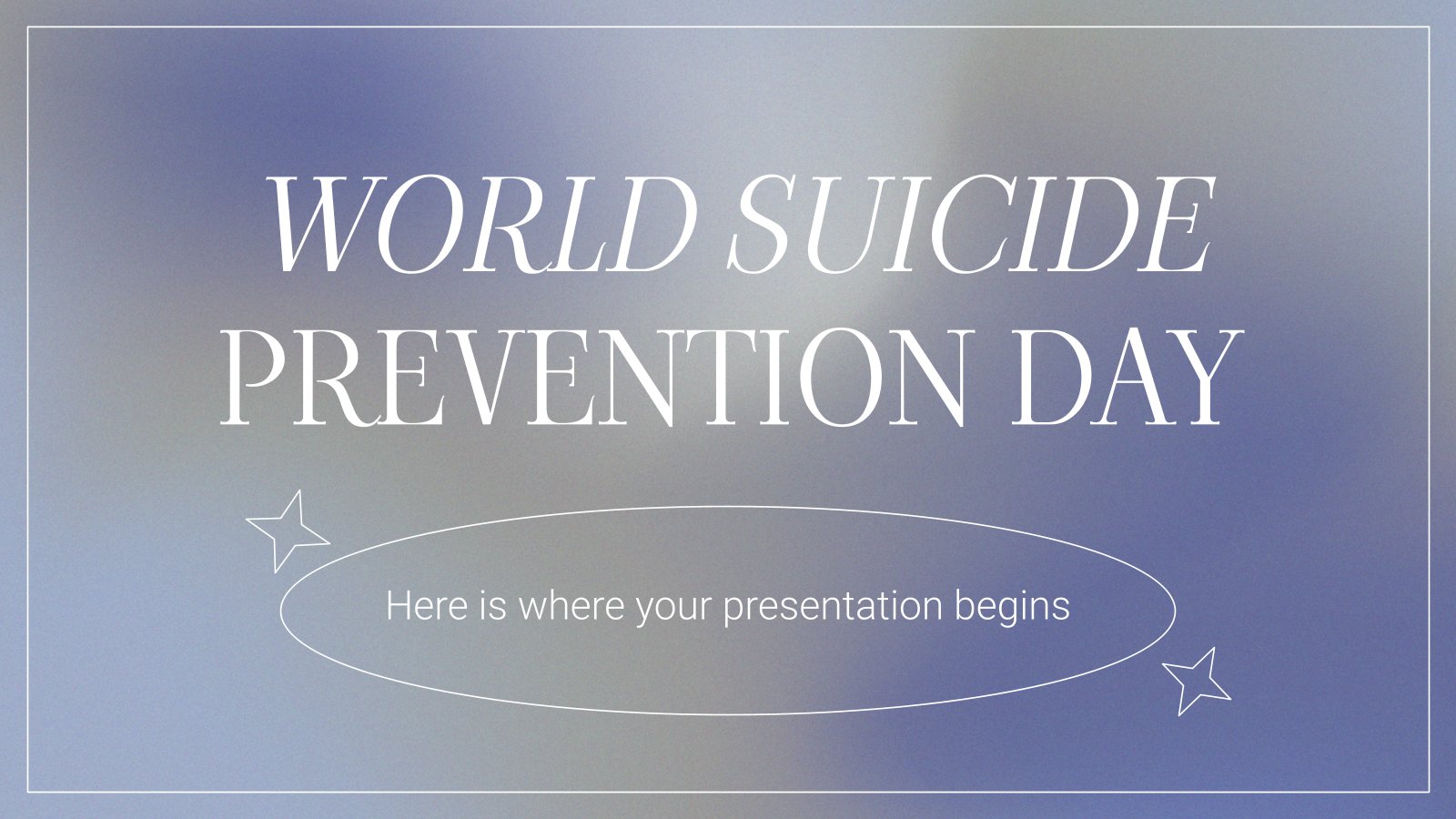
suicide prevention
9 templates
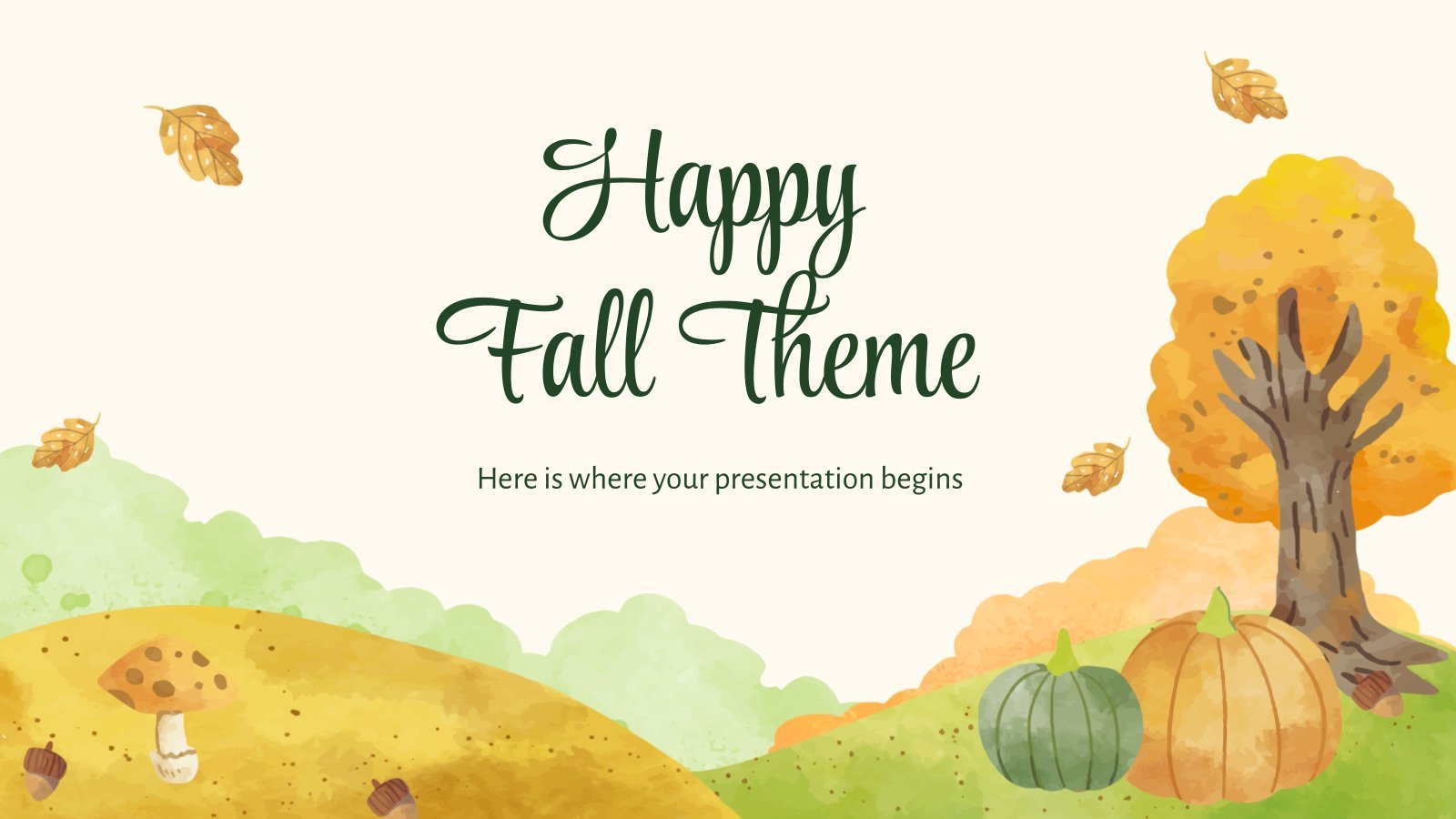
Renewable and Non-renewable Energy
It seems that you like this template, renewable and non-renewable energy presentation, free google slides theme, powerpoint template, and canva presentation template.
Our dependency on fossil fuels and non-renewable energy sources will sooner or later come back to bite us. The planet is speaking in a dangerous manner: climate change. Isn't there something we can do about it? Yes! We can rely on renewable energy, reducing pollution and guaranteeing an "indefinite" supply. The sooner we're aware of this, the better! Teach about renewable and non-renewable energy in school, and who's a better ally in education than Slidesgo? We've prepared this template, with real content by educators, some photos and colorful gradients, to make things much easier for you. This template is available in different languages, so enjoy!
Features of this template
- Designed for teachers and parents
- 100% editable and easy to modify
- 21 different slides to impress your audience
- Contains easy-to-edit graphics such as graphs, maps, tables, timelines and mockups
- Includes 500+ icons and Flaticon’s extension for customizing your slides
- Designed to be used in Google Slides, Canva, and Microsoft PowerPoint
- 16:9 widescreen format suitable for all types of screens
- Includes information about fonts, colors, and credits of the resources used
- Available in different languages
How can I use the template?
Am I free to use the templates?
How to attribute?
Attribution required If you are a free user, you must attribute Slidesgo by keeping the slide where the credits appear. How to attribute?
Available in.
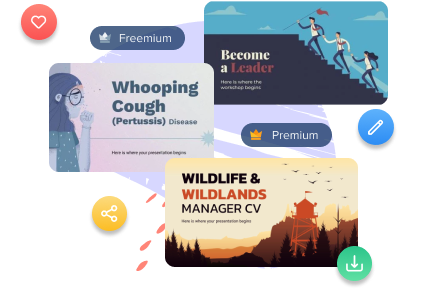
Register for free and start downloading now
Related posts on our blog.
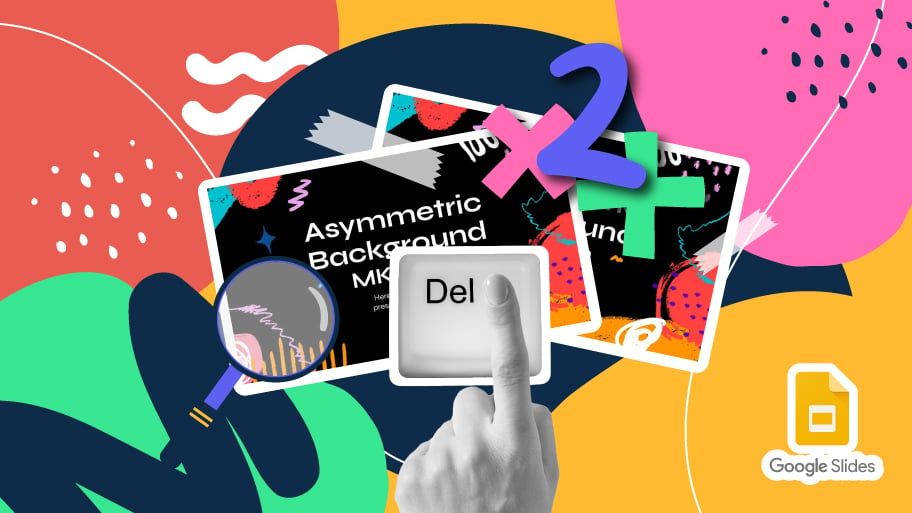
How to Add, Duplicate, Move, Delete or Hide Slides in Google Slides

How to Change Layouts in PowerPoint
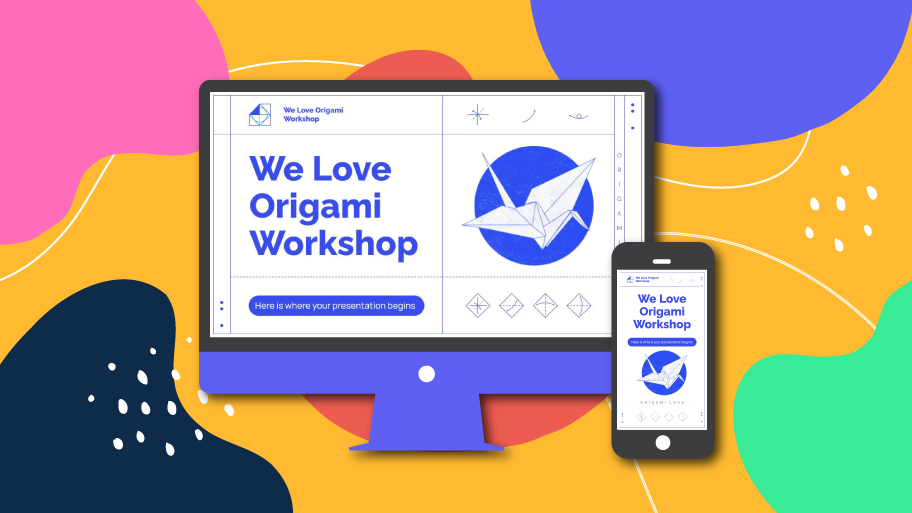
How to Change the Slide Size in Google Slides
Related presentations.
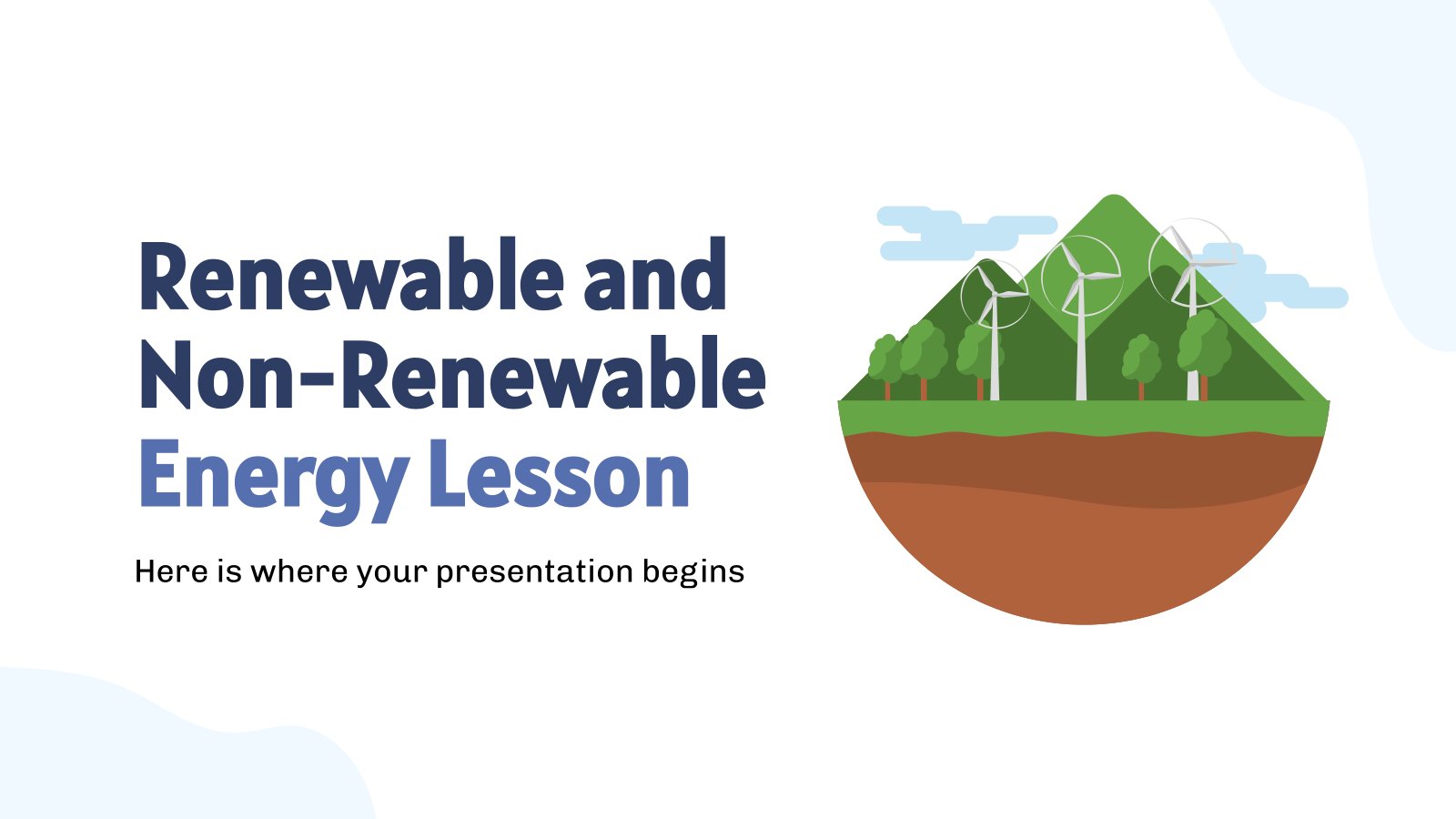
Premium template
Unlock this template and gain unlimited access
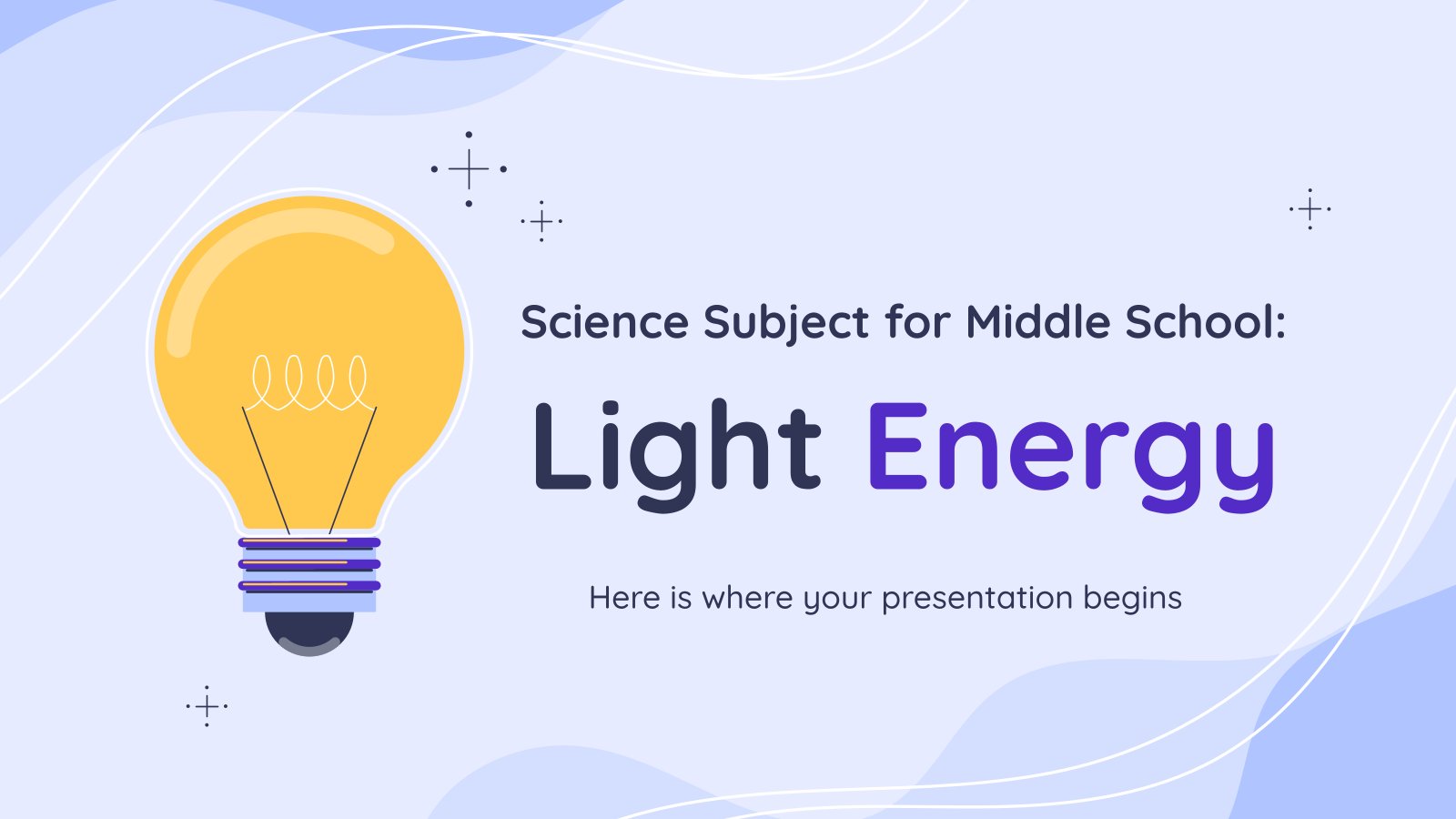
- ORIGINAL ARTICLE
- Open access
- Published: 06 March 2017
A case study of a procedure to optimize the renewable energy coverage in isolated systems: an astronomical center in the North of Chile
- H. Abos 2 ,
- M. Ave ORCID: orcid.org/0000-0001-7386-4828 1 &
- H. Martínez-Ortiz 2
Energy, Sustainability and Society volume 7 , Article number: 7 ( 2017 ) Cite this article
11k Accesses
3 Citations
Metrics details
Renewable energy resources show variabilities at different characteristic time scales. For a given resource and demand pro le, there is an absolute maximum achievable coverage (when limiting the fraction of energy lost during production and delivery to a reasonable value). To reach larger coverage factors, two plausible paths can be taken: a mix of resources with different time variabilities and/or an energy storage system. The case treated in this paper is the electricity supply of an Astronomical Center in the North of Chile. The economical feasibility of both possibilities is explored and compared to a grid connected alternative.
First, data from local weather stations was collected to have a realistic evaluation of the variability of the solar/wind resource at all time scales. Then, we developed a scalable design of a solar/wind plant and a pumped hydro energy storage system. The free parameters of the design are the maximum installed power for each resource and the capacity of the storage system. Finally, the electricity production is calculated to determine the coverage factor and losses for different values of these parameters.
We found that a coverage factor of 64% is economically feasible for systems without storage. The associated total losses are 24%. To reach larger coverage factors is not economically possible and a storage system must be introduced. If this is done, there is a quantum increase of the total cost of about 30%. However, losses are reduced to about 5% and the coverage factor reaches almost 90%. The cost increase is marginally economically feasible, but it has some other advantages: the consumer is independent of the volatility of electricity prices, and is more sustainable.
The time variability of renewable energy resources difficults reaching coverage levels larger than 60%. Energy storage systems are a requirement. Periods of zero net production seem unavoidable unless the renewable energy and storage system are largely overdimensioned. Back up systems based on fossil fuels seem to be unavoidable. Both the energy storage and back up system add an extra cost that has to be paid if such high coverage levels are a requirement.
The case treated in this paper is the electricity supply of an Astronomical Center in the North of Chile. The ESO is the European Organization for Astronomical research in the Southern hemisphere. It operates the VLT (very large telescope), located at Cerro Paranal in the Atacama desert, North of Chile. The E-ELT (European extremely large optical/infrared telescope) in Cerro Armazones (20 km away from Cerro Paranal) is in advanced design phase and will be the largest optical telescope in the world. Finally, the CTA collaboration (Cherenkov Telescope Array) has chosen the Armazones-Paranal site for construction of its Southern Observatory.
When the two new observatories enter in operation, the peak power demand of the Armazones-Paranal site is estimated to be ∼ 8.5 MW and the total annual energy consumption ∼ 70 GWh. Currently, the VLT is generating its own electricity using fossil fuel-based generators.
The two main characteristics of this consumption center are the strong requirements on the stability of the electricity supply, and the relatively large power demanded. Due to these two factors, the use of liquid fossil fuels is economically un-viable. The only two non-renewable solutions plausible are connection to the Chilean national grid or self production of electricity using generators run with natural gas from a nearby pipeline.
The main renewable energy resources available at the site are wind and solar. In this work, we consider a wind-solar PV plant with Pumped Hydro Energy Storage (PHES). We calculate the coverage factor for different values of total Power, Maximum Energy Storage and wind to solar fraction to find energy systems that maximize coverage but with costs below the non-renewable energy solutions. Embedded in this procedure is the fact that renewable energy time variability can be diminished by considering a mixture. An important ingredient of this procedure is the relative cost of each technology. Government estimates are taken when possible.
Additionally, a concentrated solar power (CSP) plant with thermal energy storage is analyzed. This technology is considered separately since the storage system cannot be used by the wind farm.
The design of the systems is not detailed but all sources of inefficiencies are taken into account. The wind and solar input data used is from local weather stations, which provides realistic time series that account for all possible sources of the variability of the resources. Overall, the estimates of electricity production and cost are as realistic as possible so they can be used as a guide if such energy systems are eventually implemented. The total cost of each system includes operation and maintenance over the 25 year lifetime of the astronomical center.
The paper is organized as follows: in “ Energy demand ” section, the energy demand is described; in “ Non-renewable energy systems ” section, the non-renewable energy systems and their cost are analyzed; in “ Renewable energy resources available in the site: solar and wind ” section, the solar and wind data used in our calculations is described; in “ Renewable energy systems ” section, the methodology to calculate the time series of electricity production for the Wind-Solar PV plant with PHES is presented, together with a modular design of each of the subsystems and their cost; in “ Results ” section, an algorithm to find the optimum system is presented and compared to the non-renewable energy alternatives. The CSP with thermal storage design and cost are presented in the Appendix .
Energy demand
The energy demand of the VLT is known [ 1 ]. The power demand changes from day to night but is rather constant along the year (less than 5% variability). The projected E-ELT (CTA) consumption is taken from ESO estimates [ 2 ]. All the sub-systems, including lodging, offices and workshops are included. A simplified model is adopted: a constant power with different day/night values. The start/end for day/night will be calculated using the sunrise and sunset, even though the start/end of astronomical observations is typically later/earlier.
Table 1 shows a summary of the site energy demand. Night consumption is smaller than day for the E-ELT and VLT due to the strict thermal control system.
All observatories work in slow tracking mode during the night. In between observational windows, telescopes are re-positioned to track new objects. The instantaneous power required for re-positioning is large compared to the average power: 700, 3200, and 2000 kW for the VLT, CTA, and E-ELT compared to 1000, 2750, and 4250 kW. However, the total energy for repositioning is small (<5%). The extra power for repositioning can be supplied by energy storage systems with extremely fast responses like flywheels, STATCOMs or a battery system.
Non-renewable energy systems
Connection to the chilean electrical network.
The grid connection alternative envisages the connection of the Armazones-Paranal site to the Paposo substation. It requires the construction of a ∼ 60 km 66 kV line, one 220–66 kV transformer (at Paposo) and one 66–23 kV substation (located halfway between Cerro Armazones and Paranal). The projected investment cost or CAPEX is 12.5 MUSD ( ∼ 11 M e ). The OPEX is calculated multiplying the total annual energy consumed (70 GWh) by a nominal price. Two cases are considered: no inter-annual increase and 1% inflation.
The electrical network in Chile consist of four independent networks, the two most important being Sistema Interconectado Central (SIC) and Sistema Interconectado del Norte Grande (SING). The electricity market is liberalized but there is a distinction between regulated ( P < 2000 kW) and special clients ( P > 2000 kW). Special clients can negotiate directly electricity prices with the producers and/or produce its own energy. Regulated clients are subject to prices fixed twice a year by the government based on the liberalized market prices. Figure 1 shows the time evolution of the mean market price in Chile for the SING/SIC in Chilean Pesos per kWh and e per MWh [ 3 ]. Prior the 2007 crisis, prices were around 40 e /MWh, and during the last 5 years have been stable around 80 e /MWh with 15% oscillations. This is the nominal price that will be considered in this work.
Time evolution of the mean market electricity price in Chile for the SING/SIC in Chilean Pesos per kWh and e per MWh
Multifuel generators
A 8.5 MW combined cycle gas turbine (CCGT) is considered in this case: it has high efficiencies ∼ 55% and fast time responses. Since there is already a 2.5 MW generator with these characteristics in the site, it will be only necessary to upgrade it with 6 MW more. We consider an investment cost of 1000 e /kW, i.e., a CAPEX of ∼ 6 M e . Natural Gas supplied by Gas Atacama, whose pipeline passes through the middle of the Armazones-Paranal site, can be used to run these generators. The expected connection cost is ∼ 2.5 M e : a gas sub-station, a low capacity (7000 m 3 per day) 5-km pipeline and a low capacity tank for regulation. In total, the CAPEX of the back-up system is 8.5 M e .
The OPEX is mainly due to the purchase of natural gas. The natural gas prices are high in Chile. The projections from the Chilean government are taken to correct the world market prices to the special case of Chile. The following equation is adopted to estimate the time-dependent price of a kWh generated by CCGT:
where C gas is given by (1+ f N years )·9, N years is the number of years since 2015 and f takes into account the interannual increase of prices. We consider two values: f =0.01 and f =0.1. This equation yields 0.07 e /kWh for 2015.
Due to the strong requirements on the stability of the supply, this system is also a requirement for all renewable energy systems considered.
Cost estimation
The total cost normalized to year 0 is estimated using:
where k is the interest rate, 3%. The lifetime of the observatories and the renewable energy system is taken as 25 years. Table 2 shows the results.
Renewable energy resources available in the site: solar and wind
The Armazones-Paranal site is located in the Atacama desert, 130 km south from Antofagasta and 1200 km north of Santiago de Chile. The Cerro Paranal and Cerro Armazones have a height of 2635 and 3000 m respectively, and they are 22 km apart. The Cerro Paranal is 15 km away from the coast.
The topography in the North of Chile is dominated by the Central Andes, characterized by four topographical segments from West to East: the coast mountain range, the central hollow, the pre Cordillera, and the Cordillera. The Armazones-Paranal site is located in the coast mountain range, 20–40 km wide and with mean heights of 1500–2000 m. The coast mountain range falls rapidly into the sea with active segments of sea abrasion where sea cliffs are present and inactive segments where there is an emerged platform.
The climate is typical of a desert region: day/night thermal differences of up to 10 o C , rainfall smaller than 30 mm and relative humidities in the 5–20% range. The average temperature is ∼ 15 with ∼ 5 o C seasonal variations.
The solar resource
The solar resource is characterized using the 2011 data from a weather station installed in the area [ 4 ]. The measurements available are global and diffuse irradiance in horizontal plane and one axis tracking mode (North-South orientation), temperature. Only 25 days have missing measurements. This data is directly used in the estimation of the electricity production of a solar based renewable energy system. This data contains all sources of time variability and in that sense is more suited for our purpose than satellite based models.
In some special cases, e.g., for missing data periods or to evaluate the inter-annual variability of a wind-solar plant, a simplified model of solar irradiance is used:
where I G is the global solar irradiance incident on a surface that subtends an angle Φ with the sun direction, f d is the fraction of diffuse irradiance and I D is the direct irradiance in the sun direction:
where θ s is the solar zenith angle, I 0 the irradiance when the sun is in the zenith and τ is an atmospheric extinction parameter. Adopting f d =0.05, I 0 =1200 W/m 2 1 and τ =0.1, a good description of the data is found. 2
Figure 2 shows the global irradiance incident on a horizontal and a one-axis tracking surface from data (dashed lines) compared to the model (solid lines) for the 23rd of June 2011. Figure 3 shows the same for the accumulated day irradiance. 34 days out of the 340 analyzed has a predicted irradiance 10% larger than measured (“Cloudy Days”) but only 5 are consecutive.
Global irradiance incident on a horizontal and a one-axis tracking surface from data ( dashed lines ) compared to the model ( solid lines ) for the 23rd of June 2011
Same as Fig. 2 but for the accumulated day irradiance
The temperature is also an important factor that determines the performance of solar plants. The weather station temperature time series is used in our calculations.
The wind resource
Wind and speed direction from the VLT meteo mast is used to characterized the wind resource [ 5 ]. Measurements at 10 and 30 m from the last 15 years exist. Table 3 shows the average wind speeds at 30 m for the last 10 years. Figure 4 shows the wind speed distribution for the year 2011 at 30 m.
Wind speed distribution at 30 m in Cerro Paranal for the year 2011
Renewable energy systems
In this section, the methodology to calculate the time series of electricity production for the wind-solar PV plant with PHES is presented. Then, a modular design of each of the subsystems is described. Finally, the procedure to calculate the cost given any value of installed power, wind to solar fraction and size of the storage system is described.
Electricity production time series: methodology
The following definitions will be adopted:
P P ( t ) MW : time series of power produced by solar/wind plant.
P D ( t ) MW : time series of power demand.
P A ( t ) MW : time series of power available to satisfy the demand (either from wind/solar plant or storage system).
E S and E MSC MWh: storage level and maximum storage capacity.
P to store and P \(_{max}^{to~store}\) : power to store and maximum instantaneous power that the storage system is able to store.
s 1 /s 2 : efficiency of the storage system to store/deliver electricity. It can depend on load.
t 1 /t 2 /t 3 : transport efficiencies (transformer and lines) between solar/wind plant-storage system (t 1 ), storage system-demand site (t 2 ) and solar/wind plant-demand site (t 3 ). t 1 /t 2 /t 3 depends on the location of each subsystem and transmission line type. For our case and using standard calculations they are: 97, 97.5, and 98%.
Time series are calculated in 10 min intervals. If P P > P D energy is stored with efficiency s 1 × t 1 , unless P to store > P \(_{max}^{to~store}\) or the storage system is full. If P P < P D energy is extracted from the storage system with efficiency s 2 × t 2 until depleted. The efficiency t 3 is also applied to the fraction of P P that directly satisfy the demand.
E \(_{loss}^{Stg}\) accounts for the energy lost because of P \(_{max}^{to~store}\) and E MSC . E \(_{loss}^{Eff}\) accounts for losses due to s 1 / s 2 . E \(_{loss}^{Transport}\) accounts for losses in transport. E \(_{loss}^{Avail}\) accounts for availability: it is included assuming that on the 15th day of each month all systems are stopped for maintenance (3.3%). It is only applied to the annual energy production.
E P , E D , and E A are the annual sum P P , P D , and P A . Other definitions:
f cover =E A /E D : energy coverage.
\(f_{loss}^{Stg}\) = E \(_{loss}^{Stg}\) /E P : energy loss due to storage size and storage maximum power.
\(f_{loss}^{All}\) =( E \(_{loss}^{Stg}\) + E \(_{loss}^{Eff}\) + E \(_{loss}^{Transport}\) + E \(_{loss}^{Avail.}\) )/ E P : total energy loss.
Solar PV plant
We present a modular design of a solar PV plant. The unit cells corresponds to ∼ 1 MWp. The components of the Solar PV plant selected are the following:
Solar panels: Jinko Solar JKM300M. This is a silicon poly-crystalline 300 Wp panel. These modules have the IEC61215 certification which is the standard in Europe.
Inverter/transformer: the Sunny Central SC1000MV. This is a central inverter optimal for large system where production is uniform across the array
Trackers: the ExoSun ExoTrackHZ, suitable for large plants deployed in flat areas. This is a one axis tracker (axis orientation North-South).
The number of panels to be placed in series is calculated using: N series = V op,inv / V mpp,panel , where V op,inv is 450–820 V and V mpp,panel is 35–40 V depending on irradiance. This gives between 11 and 23 panels per string . The open circuit voltage of a string ( N series x45 V) should not exceed the maximum operating voltage of the inverter (880 V). For that reason 18 panels per string are chosen. 30 string s will be connected to a tracker forming a block , fulfilling the tracker specifications. All strings within a block are connected in parallel to an inverter. The number of blocks to be connected in parallel to reach the nominal inverter power is given by \(\frac {P_{inverter}}{N_{blocks}\times 30 \times 18 \times P_{nom,panel}}\) . This yields six blocks per inverter, which also complies with the restriction that the short circuit current does not exceed the maximum allowable current of the inverter.
Each string is a 2 x 18 m rectangle. 30 of them are placed consecutively (with a spacing of 7 m) to form a block. The spacing is chosen to minimize shading losses. 3 x 3 blocks are placed side by side to minimize DC cabling forming a unit cell, a rectangle of ∼ 280 x 64 m.
The power produced by the solar PV plant in a given time period is given by:
where I G ( Φ ) is the global solar irradiance on a surface with an incidence angle Φ , I stc the irradiance in standard conditions 1000 W/m 2 , the factors f therm . and f shading take into account the thermal and shading losses that depend on irradiance, ambient temperature and sun position, the factor f cte are losses that have no dependencies on the time period considered. The angle Φ is calculated for each period so the solar vector lies within the plane perpendicular to the aperture. The only exception is when the required solar panel elevation is smaller than what trackers allow (40 o , since trackers can rotate ±50 o ). In that case, the incidence angle is calculated for a fixed elevation of 40 o .
The thermal losses are calculated using:
where g is the thermal losses coefficient (0.4% per o C ), T std is the temperature in standard conditions (25 o C ) and T panel is the panel temperature that can be calculated using:
where T c is the characteristic temperature of the panel, 45 o C in our case, and T ambient is the ambient temperature taken from the weather station.
The shading losses are estimated by geometric calculations for each time period considered. The constant losses are 7%, see Table 4 .
Panel degradation is 20% over 25 years. Only the production of the first year is calculated. To maintain it over 25 years, extra power will be deployed that will be accounted in the OPEX of the plant.
Using the meteo-mast data and a topographic map of the area, we followed the standard procedure to design a wind farm. The software WASP is used to generate a wind resource map (WRG), see Fig. 5 . Then, the OpenWind Software is used to design wind farms with two, five, and ten turbines. The location is 15 km to the west of Cerro Paranal in the Coastal Cliff, where the wind power density is the highest. The wind turbine chosen is the Alstom ECO 80 2.0 Class 2. It is a pitch regulated 2 MW wind turbine, with a hub height of 80 m, a cut-in wind of 4 m/s and a cut-off wind of 25 m/s.
Map of the wind power density to the west of Cerro Paranal. The wind power density is higher in the pink areas . We also show the wind direction rose at the location of the Cerro Paranal. The areas with high wind density on the left correspond to the Coastal Cliff, about 15 km away from the Cerro Paranal
The mentioned software does not provide a time series of the produced electricity. This is a problem for our study: an storage system cannot be dimensioned without them. To overcome this problem, we use the following assumption to characterized the time series:
where P Turbine is the turbine power as a function of air density and wind speed at hub height:
where v 30 ( t ) is the measured temporal series of the meteo mast at 30 m, f vertical is a factor to extrapolate measurements to different heights:
and f horizontal is a factor that takes into account the geographical variations of the wind speed. The value of f horizontal is adjusted so Eq. 8 gives the same duty factor as OpenWind.
The PV and Wind plant requires an electricity based storage system that fulfills the following criteria:
Power: ∼ 10 MW.
Discharge time at output power: more than 12 h.
Response time: ∼ 10–30 min.
Lifetime 25 years.
Efficiency: high, at least 75%.
Technologically mature.
The only technology that matches these criteria is the pumped hydro energy storage (PHES). The site is located in the Atacama desert where water is scarce. Due to the proximity to the coast, there is the possibility to use sea water as storage medium. However, due to the size of the facility and plausible technological and environmental problems, it is advised the use of desalinated water either self produced or bought.
The PHES plant consist in an upper and lower water reservoir connected by penstocks, and a system of turbines and pumps than convert gravitational energy into electricity or vice versa. The system is closed, so filling of the reservoirs has to be done only once. A separate turbine and pumping system is planned, so typical elapsed times to go from pumping to full load generation are of the order of minutes. Water evaporation 4 and filtration of water are important and will be taken into account in the design. P \(_{max}^{to~store}\) is fixed to 14 MW, so hydraulic losses does not severely affect the design.
The hydro power in W is given by:
where ρ is the water density in kg/m 3 , g is the gravity acceleration constant in m/s 2 , Q is the water flow rate through the penstocks in m 3 /s, and δ h n is the net height difference given by:
where δ h g is the gross height difference and δ h ( Q ) are the hydraulic losses in the whole system that depend on the flow rate. The electric power in generation mode is given by:
where η turb and η gen is the efficiency of the turbine (that depends on load) and the generator. The electric power in storage mode is given by:
where η pump and η mot is the efficiency of the pump system (that depends on load) and the motor.
The required value of P e turb / P e pump is 8.5/14 MW.
The design of the system proceeds in two phases:
Site selection.
Plant design.
The site selection implies indirectly choosing two important variables: δ h g and penstock length. The second variable is crucial when determining the hydraulic losses, and is an important contributor to the total cost of the system. As a general rule, larger values of δ h g and smaller penstock length yield smaller investment costs. However, other factors have been analyzed:
Existence of infrastructures like roads and transmission lines.
Existence of hydro resources or possibilities to obtain them.
Earthquake risks.
Detritus removal: short but intense rainfalls can generate detritus removal that can affect the integrity of the PHES.
Topographic maps have been used to choose four possible sites. All sites have similar availability of water/infrastructures and geological risks. Therefore, the site with larger height difference and the smaller penstock length was chosen. Figure 6 shows a detailed topographic map of the site. It is located in the Coastal Cliff, close to the Wind Farm location.
3D map of the selected PHES site together with the elevation contour
Our choice for the turbine system is the use of two Pelton turbines with one injector that can work in parallel to provide the maximum power. The Pelton turbines can work up to 10% of the nominal load, have efficiencies around 90% and are adequate for the site height differences and required nominal flows. The turbines will be coupled to two generators with nominal power 5 MW, AC output voltage of 6 kV and 98% efficiency.
Regarding the pumping system configuration, our choice is the use of multistage centrifugal pumps: 6 of 2 MW and 2 of 0.5 MW. To simplify the calculations an efficiency of 90% for all loads is considered. The motors that drive the pumps work at 6 kV with an efficiency of 98%.
Steel penstocks have rugosities of ∼ 0.6 mm. The hydraulic losses are calculated using standard formulas for different pipe diameters. For each case, the nominal flow rate in production and storage mode is calculated by solving iteratively Eq. 13 /Eq. 14 . The hydraulic losses drop below 5% in both modes at nominal conditions for a tube diameter of 0.85 m. Losses because of other hydraulic components like valves, bypasses, contractions/expansions, etc. are small (10% of Penstock losses) and taken into account. Table 5 gives the final nominal flow rate and hydraulic losses in both modes. Using these calculations the storage efficiencies s 1 and s 2 are calculated.
The penstock wall thickness required to withstand the hydrostatic pressure is given by:
where e s is extra thickness in meters to allow for corrosion, k f is the weld efficiency (0.9), D is the pipe diameter in meters, σ f is the allowable tensile stress in Pascals (1400 kgf/cm 2 , i.e., 1.373 10 5 Pa) and P is the hydrostatic pressure in Pascals. Since the hydrostatic pressure changes from the upper to the lower reservoir, the penstock is built in sections of 100 m with decreasing thickness (10–30 mm). The total weight of the penstock is ∼ 1000 tons.
The surge pressure for the water-hammer effect at the pumping nominal load is 450 m, which would require a substantial increase of the thickness walls that would yield to a doubling of the total penstock weight, i.e. its cost. For that reason, the installation of a surge tower or relief valves is necessary.
The free parameter of the design is the maximum storage capacity, E MSC MWh. For a given value of E MSC , the volume of the water reservoirs is calculated by multiplying the flow rate in generation mode by E MSC /8.5 h, adding a 20% safety margin. In the selected site, there is room for reservoirs with storage capacities up to 1000 MWh.
The reservoirs will be constructed following the scheme of an Earth/Rock filled dam. The depth of the reservoir will be 14 m, leaving 1.3 m between the maximum water level and the top of the dam. The digged material will be reused to build the trapezoidal perimetral dike (3:1), which fixes the dimensions of the reservoir. The surface in contact with the water and the air-water layer is covered by a geotextile cloth.
To build and maintain the upper reservoir it is necessary to construct a 12 km access road. In the case of the lower reservoir there is a nearby access road, so only a short and flat connection to it is necessary. It will be also necessary to build a housing for the electromechanical equipment.
The total cost after 25 years of the wind-solar PV plant with PHES storage is estimated using Eq. 2 . The CAPEX in that equation has the following components:
Solar PV and wind plant: total power installed times a unitary cost of 1,700 e /kW.
PHES: the cost of a PHES system with E MSC =110 MWh is estimated to be 26.4 M e . Table 6 shows a breakdown. The PHES cost for different values of E MSC is estimated using:
where C 1 =3.8 M e is the baseline cost of water and reservoir and C 2 =19.51 M e is the cost of the rest of the subsystems.
Back up system: 8.5 M e .
Electrical infrastructures: 3.5 M e , see Table 7 .
The OPEX has the following components:
Insurance and O&M: we assume 2% of CAPEX with 3% inter annual increase.
Gas purchases: (1− f cover )·70 GWh times the unitary price given by Eq. ??.
Solar PV: the required annual enhancement of the power installed to reach the same nominal production as the first year 5 .
The electricity production is simulated for the following parameters:
P total MW : 10, 12, 14, 16, 18, 20, 22, 24, 26, 28, 30, and 40.
f solar : 0, 0.25, 0.5, 0.75, and 1.
E MSC MWh: 0, 20, 40, 60, 80, 100, 120, 240, 480, 1000.
An example of the time series is shown in Fig. 7 for P total =20 MW, f solar =0.5 and E MSC =100 MWh. For each simulated case, the annual value of f cover and \(f_{loss}^{All}\) is calculated. The left (right) panel in Fig. 8 show an example: f cover ( \(f_{loss}^{All}\) ) as a function of the total installed power for E MSC =0 MWh and three cases of f solar , 0, 0.5, and 1. For this value of E MSC , the optimum system in terms of coverage is neither purely wind or solar, but a mixture. \(f_{loss}^{All}\) for small P total is due to availability and transport.
An example of the time series of electricity production for P total =20 MW, f solar =0.5 and E MSC =100 MWh
f cover and \(f_{loss}^{All}\) as a function of the total installed power for E MSC =0 MWh and three cases of f solar , 0, 0.5, and 1
On the basis of the costs shown in Table 2 , we select two target maximum costs: 100 and 130 M e . For each simulated case, the total cost over 25 years is calculated as in “ Cost estimation ” section. The case with a cost below the target and with maximum coverage is kept. The two cases selected for the two targets are shown in Table 8 . The coverage factors are as large as 64 and 88%. It should be mentioned that the losses for the high cost target are driven by the storage efficiency, transport losses, and availability.
Finally, the case of a concentrated solar power (CSP) plant with thermal energy storage is analyzed. This technology is considered separately since the storage system cannot be used simultaneously by the Wind farm 6 . The design and costs are presented in Appendix . The total cost is 124 M e and f cover 72.5%. This alternative is within the high cost target, but it has lower coverage factor than the case presented in this section.
1 I 0 is only 12% smaller than the irradiance outside the atmosphere (1370 W/m 2 ), which is an indicator of the quality of the site.
2 The electricity production using the model and the raw data for the reference year agrees within 5%.
3 α =0.08 from the ratio of the measurements at 10 and 30 m. A conservatively smaller value is taken: measurements at 10 m can be affected by the surrounding buildings
4 According to our estimations, it can be severe, reducing the water level by almost 3 m per year.
5 It is calculated assuming: PV system prices will decrease at a rate of 20% over 25 years; PV module degradation is 20% over 25 years.
6 Electricity from the Wind Farm would have to be converted into thermal energy. To convert back to electricity the efficiency is given by the steam turbine, ∼ 32%.
Concentrated solar power (CSP) plant with thermal energy storage
The CSP is a technology that needs to be considered when there is plenty available land, the cloudy fraction is small and the fraction of direct irradiance is high. The dessert characteristics of the site fulfill these three criteria. The technology considered in this work is the parabolic trough collectors (PTC), widely considered in a stage of maturity.
In a CSP plant, an oil is heated in the solar field from 293 o C to 393 o C and sent either to the thermal storage system or to a heat exchanger that produces water vapour at 380 o C and 104 bar. The vapour is then conducted to a steam turbine coupled to a generator. After the turbine, the vapour is taken to a condenser and fed again into the loop. Due to the scarcity of water in the site, aerocondensers are considered. The efficiency to convert thermal energy into electricity depends on the nominal power of the turbine and for a 10 MW steam turbine is ∼ 32%.
The solar field is an array of PTCs. The mirrors have a one axis tracking system (North-South) that ensures that at all moments the solar vector lies within the plane perpendicular to the aperture of the collector. Alignment is a strong requirement in PTCs, and also cleaning.
The PTCs have lengths between 100 and 150 m. The 8 module EuroTrough collector with PTR-70 Schott tubes is selected. N series of these modules are placed in series to form a group. N parallel groups are connected in parallel in central feeding configuration to minimize pipe lengths. The separation between rows of collectors is three times the width of the parabola to ensure that annual shadowing losses are below 1%.
The thermal power captured by the collector is given by:
where \(\eta _{opt \phi =0^{0}}~K(\Phi)\phantom {\dot {i}\!}\) is a parameterization of the optical and geometrical losses of the collector, A c is the aperture area, I D is the direct irradiance in W/m 2 at the period considered, F e is a factor that takes into account the dirt in the mirrors (0.95), and P losses are the thermal losses parameterized with its dependence on the temperature difference between the fluid and the ambient, as well as on the direct irradiance and incidence angle.
The collected power can also be written as:
where Q m is the fluid mass flow in kg/s, C p is the specific heat in J/K Kg and T in /T out is the start/final temperature of the fluid. The thermal fluid chosen is an oil called Therminol VP1. Its maximum working temperature is 398 o and solidification temperature is 12 o . This fluid has to be pressurized to 10.5 bar so it is not gas phase at the maximum working temperature. The specific heat and density depends on temperature and is taken from a parameterization provided by the manufacturer.
N series of collectors have to rise the fluid temperature from T in =293 o C to T out =393 o C. The necessary value of Q m is calculated iteratively by equating Eqs. 17 and 18 in 1 m intervals.
The fluid must circulate in a regime turbulent enough to avoid thermal gradients between the external/internal face of the tube that can cause fractures. The optimum value of N series is calculated by imposing a condition on the Reynolds number of the circulating fluid for the time of maximum direct irradiance. In our design, N series must be 4.
The hydraulic losses are calculated for each configuration of the system ( N series , N parallel ) and time period considered using the oil and tube characteristics and ambient conditions. Losses in the pipes that connect the collectors with the heat exchanger and the losses in the pump are also taken into account. 7 .
The required electrical pumping power is given by:
where η m ( ∼ 70%) and η e ( ∼ 99%) are the mechanical and electrical efficiency of the pump.
The electrical power produced by the plant is given by:
where η is the efficiency to convert thermal to electrical energy (32%). The storage efficiencies considered are s 1 = s 2 =96% (Round trip efficiency of 92%). Transport losses are only applicable to t 3 (2%). The availability is included as described before.
The electricity production described in “ Electricity production time series: methodology ” section is calculated in 10 min intervals during a period of 48 hours around the summer solstice. N parallel is increased until f cover =100%. The required value of N parallel is 33. E MSC is given by the maximum storage level during the design period (100 MWh).
The storage system must be able to store 100 MWh, i.e., 312 MWth. This capacity is increased by a safety margin of 8%, i.e., 337.5 MWhth. The temperature in the hot/cold tank corresponds to the temperature of the oil before/after the heat exchanger. Nitrate salt (60% by weight NaNO 3 and 40% KNO 3 ) is considered as storage medium. The mass required can be calculated using:
which yields 8530 tons of salt to store 337.5 MWth. The corresponding volume of the hot and cold tank is different due to temperature. The volume required for the cold/hot tank is 4471 and 4618 m 3 . Fast fluctuations of the solar resource are easily tracked by the thermal storage system by controlling the flow from the solar field that is diverted to the heat exchanger of the storage system.
The electricity production is then calculated for the whole year. The results are shown in Table 9 together with the main design parameters.
A flat area is necessary to ease installation of the solar field. A possible site has been found 10 km away from Cerro Paranal. The access road to the Cerro Paranal passes by the solar field, so no extra civil works are planned. For electrical infrastructures and their cost, see Table 10 .
The investment cost (CAPEX) of the CSP plant is estimated to be 58.5 M e . Table 11 shows the breakdown. The OPEX considered is 2% of the CAPEX with a 3% inter annual increase. The total cost after 25 years normalized to year 0 is 124 M e .
Abbreviations
British thermal unit
Capital expenditure
Combined cycle gas turbine
- Concentrated solar power
Cherenkov telescope array
European extremely large telescope
European southern observatory
Million US dollars
Open software to design Wind Farms
Operating expenditure
Pumped hydro energy storage
- Photovoltaic
Parabolic Trough Collectors
Sistema interconectado central
Sistema interconectado del Norte Grande
Static synchronous compensator
Very large telescope
Wind energy industry-standard software
Wind Resource Map (Power density)
Interest rate
Global solar irradiance in a surface W/m 2
Direct irradiance in the sun direction
Diffuse irradiance in a surface expressed as a fraction of the direct irradiance
Angle subtended by the normal of a surface with the sun direction
Solar zenith angle
Atmospheric extinction parameter
Time series of power produced by solar/wind plant in MW
Time series of power demand in MW
Time series of power available to satisfy the demand in MW
Annual sum P P ,P D and P A E S and E MSC : Storage Level and Maximum Storage Capacity in MWh
Power to store and Maximum Instantaneous Power that the storage system is able to store
Efficiency of the storage system to store/deliver electricity
Transport efficiencies (transformer and lines) between solar/wind plant-storage system (t 1 ), storage system-demand site (t 2 ) and solar/wind plant-demand site (t 3 )
Energy lost during storage operations due to P \(_{max}^{to~store}\) and E MSC
Energy lost during storage operations due to s 1 / s 2
Energy lost due to transport inefficiencies
Energy lost due to operation and maintenance (availability)
E A /E D energy coverage
E \(_{loss}^{Stg}\) /E P , energy loss due to storage size and storage maximum power
(E \(_{loss}^{Stg}\) +E \(_{loss}^{Eff}\) +E \(_{loss}^{Transport}\) +E \(_{loss}^{Avail.}\) )/E P , total energy loss
The irradiance in standard conditions 1000 W/m 2
Watt Peak, solar panel power for I stc
The solar panel temperature in standard conditions (25 o C )
Solar panel losses, thermal, shading and those that do not depend on solar irradiance
Solar panel thermal loss coefficient
Inverter input voltage range
Solar panel volage at maximum power
Wind speed at hub height
Wind speed height coefficient
Air density
Water density
Gravity acceleration constant
Water flow rate through the penstocks in m 3 /s
Net height difference between upper and lower reservoir in a PHES
Gross height difference
Q dependent hydraulic losses
Efficiencies of turbine, generator, pump and motor in a PHES
Hydro power
Electrical power
Penstock diameter
Length of penstock
Penstock weld efficiency
Allowable tensile stress in Pascals
Hydrostatic pressure in penstock
PTC thermal losses
PTC losses due to dirtying
Thermal power captured by a PTC
Optical and geometrical losses of the collector
Specific heat of PTC thermal fluid
Mass flow in kg/s of the thermal fluid
Temperature in/out of the thermal fluid
Towards a Green Observatory. https://www.eso.org/sci/libraries/SPIE2010/7737-73.pdf . Accessed Feb 2017.
The E-ELT construction proposal. http://www.eso.org/public/products/books . Accessed Feb 2017.
Comision Nacional de Energia de Chile. www.cne.cl. Accessed Feb 2017.
Ministerio de Energía de Chile. http://antiguo.minenergia.cl . Accessed May 2015.
European Southern Observatory. http://archive.eso.org . Accessed Feb 2017.
Download references
Acknowledgements
This work would not be possible without the financial support of the CNPq, FAPESP (PROCESSO 2015/15897-1) and the resources of the Instituto de Física de São Carlos. We thank Vitor de Souza for the careful reading of the manuscript, Eduardo Zarza for his guidance with CSP technology, Marcos Blanco for providing the WASP simulations needed to estimate the Wind Resource, Marc Sarazin for his help with the Wind data and ESO water supply, and Natalia Serre for all the information she provided concerning CTA power supply. Finally, we also thank all the Escuela de Organizacion Industrial (EOI) staff for their support.
Authors’ contributions
All authors contributed to the development of the work. The corresponding author prepared the manuscript, but all authors read and approved the final manuscript.
Competing interests
The authors declare that they have no competing interests.
Author information
Authors and affiliations.
Instituto de Física de São Carlos, Universidade de São Paulo, CP 369, São Carlos, 13560-970, SP, Brasil
EOI, Escuela de Organizacion Industrial, Madrid, Spain
H. Abos & H. Martínez-Ortiz
You can also search for this author in PubMed Google Scholar
Corresponding author
Correspondence to M. Ave .
Rights and permissions
Open Access This article is distributed under the terms of the Creative Commons Attribution 4.0 International License ( http://creativecommons.org/licenses/by/4.0/ ), which permits unrestricted use, distribution, and reproduction in any medium, provided you give appropriate credit to the original author(s) and the source, provide a link to the Creative Commons license, and indicate if changes were made.
Reprints and permissions
About this article
Cite this article.
Abos, H., Ave, M. & Martínez-Ortiz, H. A case study of a procedure to optimize the renewable energy coverage in isolated systems: an astronomical center in the North of Chile. Energ Sustain Soc 7 , 7 (2017). https://doi.org/10.1186/s13705-017-0109-0
Download citation
Received : 25 November 2016
Accepted : 10 February 2017
Published : 06 March 2017
DOI : https://doi.org/10.1186/s13705-017-0109-0
Share this article
Anyone you share the following link with will be able to read this content:
Sorry, a shareable link is not currently available for this article.
Provided by the Springer Nature SharedIt content-sharing initiative
- Pump hydro energy storage
- Applications
Energy, Sustainability and Society
ISSN: 2192-0567
- General enquiries: [email protected]

IMAGES
COMMENTS
Case study on Renewable energy - Free download as Powerpoint Presentation (.ppt / .pptx), PDF File (.pdf), Text File (.txt) or view presentation slides online.
bling higher shares of renewable energy. These case studies show that a transformation of the electricity sector towards renewables is already happen-ing, but several studies suggest that even higher shares of renewable energy power generation are foreseen. For example: The International Energy Agency's (IEA) "sus-
This work also create an outline for the presentation of combined heat and power (CHP) frameworks, notably it evades from 100 to 500 kW over a period of 20 years. ... "A Case Study on Renewable Energy Sources, Power Demand, and Policies in the States of South India—Development of a Thermoelectric Model" Sustainability 14, no. 14: 8882. https ...
It still accounts for 10% of world primary energy supply and is the world's largest single renewable energy source, since much of the world's population uses wood, charcoal, straw, or animal dung as cooking fuel (IEA 2012). Industrial economies may use biomass energy in several different forms.
Renewable energy sources play a role in providing energy services in a sustainable manner and, in particu-lar, in mitigating climate change. This Special Report on Renewable Energy Sources and Climate Change Mitigation explores the current contribution and potential of renewable energy (RE) sources to provide energy services for a sus-
In Australia, the Institute of Sustainable Futures (ISF) at the University of Technology Sydney produced scenarios to explore "100% renewable energy for Australia" (UTS, 2016). The most ambitious scenario in the report results in a renewable electricity system by 2030 and a fully renewable energy system by 2050.
PowerPoint Presentation. Ricardo Gorini, DSc. • Virtual • June 2022. Session 2: Sustainable Water and Energy Solutions & Energy Case Studies (10:15 - 13:00) Session 2: Sustainable Water and ...
Cost Savings: $300,000 (@$0.15/kWh) Cost: $12.25 million. The Housing Authority of the County of Santa Barbara (HACSB) has successfully implemented a portfolio-wide renewable energy strategy offsetting 100% of the electrical consumption at 21 properties and HACSB's administration buildings. The 1.7 mW project involved the installation of over ...
Renewable Energy Resources: Case Studies . Balaji Devarajan 1, V Bhuvaneswari 1, A K Priya 1, G Nambirajan 1, J Joenas 1, P Nishanth 1, L Rajeshkumar 2, G Kathiresan 3 and V Amarnath 3 .
transmit renewable energy into our power grid can further fragment habitats and become conduits for non-native species that disrupt ecosystems. Our attempts to meet climate change emission targets and close the energy access gap could create a new problem of "energy sprawl" that accelerates land-use change and conflict.
The case study in Montenegro highlights the effectiveness of renewable energy investments in reducing emissions, emphasising the need for cost-effective emission reduction strategies in the energy sector. Despite study limitations, these findings underscore the importance of advancing cleaner and sustainable energy systems globally, balancing ...
Energy Efficiency & Renewable Energy. Chapter 16. Core Case Study: Iceland's Vision of a Renewable-Energy Economy (1). Supplies 75% of its primary energy and almost all of its electrical energy using Geothermal energy Hydroelectric power No fossil fuel deposits: imports oil. 1.44k views • 84 slides
The greening of existing jobs is an outcome of the implementation of energy efficient green technologies. This case study refers to two projects in the energy sector, i.e. 1. Biomass Based Power Plant: This project includes the construction, installation and operation of a 6MW grid connected and biomass based power plant (rice husk). The
Case Study Publications. ECE Energy Series 58: Application of UNFC Case Studies 2019 (2019) A series of case studies on various resource projects, including niobium, oil & gas, rare earth elements, thorium and uranium, from different countries. The case studies serve to demonstrate how UNFC could be applied to assure sustainable resource ...
The term "renewable" encompasses a wide diversity of energy resources with varying economics, technologies, end uses, scales, environmental impacts, availability, and depletability. For example, fully "renewable" resources are not depleted by human use, whereas "semi-renewable" resources must be properly managed to ensure long-term ...
An overview of renewable energy sources, including (a). solar photovoltaics, (b). concentrating solar power, (c). thermophotovoltaics, (d).thermoelectrics and (e). wind mills is presented ...
Includes 500+ icons and Flaticon's extension for customizing your slides. Designed to be used in Google Slides, Canva, and Microsoft PowerPoint. 16:9 widescreen format suitable for all types of screens. Includes information about fonts, colors, and credits of the resources used.
The NPC of this. system is also very high at $416,786 which is $34,355 greater than the grid only system. The resort is too small to sustain a large wind turbine, the most efficient system using a. large turbine has a renewable factor of .94, but the NPC is very high at $1,232,837.
Biomass energy is energy produced by burning biomass. Biomass is a broad category of material encompassing all the living matter in an area. Wood, crops and crop waste, and wastes of plant, mineral, and animal matter comprise much of an area's biomass. Much of this material is considered "garbage" and ends up in landfills.
Background Renewable energy resources show variabilities at different characteristic time scales. For a given resource and demand pro le, there is an absolute maximum achievable coverage (when limiting the fraction of energy lost during production and delivery to a reasonable value). To reach larger coverage factors, two plausible paths can be taken: a mix of resources with different time ...
2. Glue tabs under oil, wind, and solar to make one long template. 3. Glue the middle two panels (biomass and wind into your notebook). Make a foldable by folding along the solid lines. Coal, oil, natural gas, and nuclear will be covered in the non-renewable lesson. . This template covers both renewable and nonrenewable energy resources.
On the other hand, in , it has been shown through a case study for a Great Britain test system that increased integration of IRESs and the location of the integration also affects the grid strength and voltage stability. ... 8760 h power curve in the case of renewable energy capacity of 426 GW.
Workfront offers robust project management capabilities, allowing managers to view team capacity, allocate resources to projects and tasks, and balance workloads across teams or individuals. It also provides tools for resource scheduling, capacity planning, and skill-based resource allocation.