- Open access
- Published: 19 May 2023

The efficacy and effectiveness of COVID-19 vaccines around the world: a mini-review and meta-analysis
- Marzieh Soheili 1 ,
- Sorour Khateri 2 ,
- Farhad Moradpour 3 ,
- Pardis Mohammadzedeh 4 ,
- Mostafa Zareie 4 ,
- Seyede Maryam Mahdavi Mortazavi 5 ,
- Sima Manifar 6 ,
- Hamed Gilzad Kohan 7 &
- Yousef Moradi 3
Annals of Clinical Microbiology and Antimicrobials volume 22 , Article number: 42 ( 2023 ) Cite this article
19k Accesses
21 Citations
183 Altmetric
Metrics details
This meta-analysis evaluated the Efficacy and Effectiveness of several COVID-19 vaccines, including AstraZeneca, Pfizer, Moderna, Bharat, and Johnson & Johnson, to better estimate their immunogenicity, benefits, or side effects.
Studies reporting the Efficacy and Effectiveness of COVID-19 vaccines from November 2020 to April 2022 were included. The pooled Effectiveness/Efficacy with a 95% confidence interval (95% CI) with Metaprop order was calculated. The results were presented in forest plots. Predefined subgroup analyses and sensitivity analyses were also performed.
A total of twenty articles were included in this meta-analysis. After the first dose of the vaccine, the total effectiveness of all COVID-19 vaccines in our study was 71% (95% CI 0.65, 0.78). The total effectiveness of vaccines after the second dose was 91% (95% CI 0.88, 0.94)). The total efficacy of vaccines after the first and second doses was 81% (95% CI 0.70, 0.91) and 71% (95% CI 0.62, 0.79), respectively. The effectiveness of the Moderna vaccine after the first and second dose was the highest among other studied vaccines ((74% (95% CI, 0.65, 0.83) and 93% (95% CI, 0.89, 0.97), respectively). The highest first dose overall effectiveness of the studied vaccines was against the Gamma variant (74% (95% CI, 0.73, 0.75)), and the highest effectiveness after the second dose was observed against the Beta variant (96% (95% CI, 0.96, 0.96)). The Efficacy for AstraZeneca and Pfizer vaccines after the first dose was 78% (95% CI, 0.62, 0.95) and 84% (95% CI, 0.77, 0.92), respectively. The second dose Efficacy for AstraZeneca, Pfizer, and Bharat was 67% (95% CI, 0.54, 0.80), 93% (95% CI, 0.85, 1.00), and 71% (95% CI, 0.61, 0.82), respectively. The overall efficacy of first and second dose vaccination against the Alfa variant was 84% (95% CI, 0.84, 0.84) and 77% (95% CI, 0.57, 0.97), respectively, the highest among other variants.
mRNA-based vaccines against COVID-19 showed the highest total efficacy and effectiveness than other vaccines. In general, administering the second dose produced a more reliable response and higher effectiveness than a single dose.
Introduction
The coronavirus disease 2019 (COVID-19) is an acute respiratory infection caused by the severe acute respiratory syndrome coronavirus 2 (SARS-CoV-2). This β-coronavirus is an enveloped, non-segmented positive-sense RNA virus, which primarily spreads through the respiratory tract [ 1 , 2 , 3 ]. COVID-19 infection is often associated with systemic inflammation and inflammatory biomarkers such as IL-6, IL-10, and TNF-α) increase in the patients [ 4 , 5 , 6 ]. Cough, fever, and shortness of breath are the dominant symptoms of COVID-19 infection. Additionally, fatigue, increased sputum production, sore throat, headache, and gastrointestinal symptoms might be observed [ 6 , 7 , 8 ]. Elderly patients with underlying disorders such as hypertension, chronic obstructive pulmonary disease, diabetes, and cardiovascular complications are more prone to develop acute respiratory distress syndrome. Other severe symptoms include septic shock, metabolic acidosis, and coagulation dysfunction, which might lead to death [ 9 , 10 ]. Various medications have already been tested for treating COVID-19 patients. However, the evidence to support the beneficial effects of these drugs is often controversial [ 11 , 12 , 13 ]. Molnupiravir is the first oral antiviral drug that has recently shown a significant benefit in reducing hospitalization or death in COVID-19 patients [ 14 ].
According to the World Health Organization (WHO) report, from the emergence of COVID-19 in December 2019 to November 2021, more than 250,000,000 confirmed cases of COVID-19 have been reported, and more than five million deaths have been attributed to the disease globally [ 15 ]. Since the COVID-19 pandemic, several studies have started to develop safe and efficacious vaccines. Numerous clinical trials have been conducted to evaluate the efficacy and safety of experimental vaccines [ 16 , 17 , 18 ]. WHO reported as of November 8, 2021, more than seven billion vaccine doses have been administered worldwide [ 15 ]. Additionally, as per the WHO report, until November 9, 2021, 130 vaccine candidates were under clinical development, and 156 candidates were in the pre-clinical development phase. Different types of COVID-19 vaccines have been developed worldwide, including protein subunit, recombinant, viral vector, RNA- and DNA-based, and sub-unit vaccines [ 19 ].
Up to now, several COVID-19 vaccines have been authorized or approved for use. WHO issued an emergency use authorization for the Pfizer COVID-19 vaccine On December 31, 2020 (BNT162b2). Next, on February 15, 2021, the Astra-Zeneca/Oxford COVID-19 vaccine (manufactured by the Serum Institute of India and SKBio) received emergency use approval, followed by Ad26.COV2.S (developed by Janssen (Johnson & Johnson)) on March 12, 2021, and Moderna vaccine on April 30, 2021 [ 20 ]. Pfizer COVID-19 vaccine is a lipid nanoparticle formulation that contains a nucleoside-modified RNA against the S protein of the SARS-CoV-2 virus [ 21 ]. Moderna is a lipid nanoparticle–encapsulated nucleoside-modified messenger RNA vaccine encoding prefusion stabilized full-length spike protein of SARS-CoV-2 (24). The Oxford/AstraZeneca COVID-19 vaccine (ChAdOx1 nCoV-19 vaccine, AZD1222) contains a replication-deficient chimpanzee adenoviral vector ChAdOx1, delivering the SARS-CoV-2 structural surface glycoprotein antigen (spike protein; nCoV-19) gene (22, 23). Janssen is a non-replicating, recombinant human adenovirus type 26, containing a full-length SARS-CoV-2 S protein [ 22 ]. Bharat (CovaxinTM) is an inactivated-virus vaccine developed in Vero cells combined with Alhydroxiquim-II (Algel-IMDG), chemosorbed imidazoquinoline onto aluminum hydroxide gel. This complex is an adjuvant to boost immune response for longer-lasting immunity [ 23 ].
Careful planning for the COVID-19 vaccination program requires comprehensive review studies to evaluate the efficacy and safety of the vaccines. This study aims to conduct a meta-analysis to assess the Effectiveness and Efficacy of COVID-19 vaccines, including AstraZeneca, Pfizer, Moderna, Bharat, and Johnson & Johnson. Well-designed meta-analysis studies will provide a more accurate overview to evaluate Efficacy and safety outcomes compared to individual studies and contribute to a better understanding of the use of the vaccine in different populations.
Materials and methods
The present systematic review and meta-analysis were conducted according to Preferred reporting items for systematic reviews and meta-analysis (PRISMA) guidelines for reviewing analytical observational studies [ 24 ].
Search strategy and screening
International databases were searched to find all original published articles, including Medline (PubMed), Web of Science, Embase (Elsevier), Cochrane Library, Scopus, Ovid, and CINHAL, to retrieve all articles evaluating and reporting the efficacy and side effects of all COVID-19 vaccine (Pfizer–BioNTech, Oxford–AstraZeneca, Moderna, Janssen, CoronaVac, Covaxin, Novavax and Convidecia) in fully vaccinated and partially vaccinated people. The studies which have compared these items with non-vaccinated individuals were also included. In addition to searching the mentioned databases, gray literature was searched by reviewing articles in the first ten pages of Google scholar. A manual search was performed by reviewing references from related studies. This search was conducted with language limitations from November 2020 to September 2022. The search protocol was developed based on four primary roots involving “vaccination,“ “COVID-19,“ “Side effect,“ and “Efficacy.“ All related components to these keywords were “vaccinated”, “non-vaccinated”, “partial vaccinated”, “fully vaccinated”, “Pfizer–BioNTech”, “Oxford–AstraZeneca”, “Sinopharm BIBP”, “Moderna”, “Janssen”, “CoronaVac”, “Covaxin”, “Novavax”, “Convidecia”, “symptoms”, “signs” (“fever”, “cough”, “malaise”, “dyspnea”, “myalgia”, “sore throat”, and “diarrhea”), “thrombosis”, “emboli”, “thromboembolism”, “thromboembolic”, which were added to the searched queries based on scientific Mesh terms, EMTREE, and Thesaurus. Reference Manager bibliographic software was applied to manage searched citations. Duplicate entries were searched by considering the papers’ title, year of publication, authors, and specifications of types of sources. In case of questionable records, the texts were compared. After reviewing the primary search results, each article was double-checked by title and available abstract, and some of the articles were omitted based on the selection criteria. The evaluation of the considered papers was based on the inclusion and exclusion criteria by the two researchers separately (SM, MS). After the screening, (YM) selected the articles by evaluating their full texts.
Eligibility criteria
We included all observational and interventional studies that assessed the Efficacy/Effectiveness and side effects of all types of COVID-19 vaccines (Pfizer–BioNTech, Oxford–AstraZeneca, Sinopharm BIBP, Moderna, Janssen, CoronaVac, Covaxin, Novavax and Convidecia) in fully vaccinated and partially vaccinated people. The studies comparing these items with non-vaccinated individuals were also included. We excluded duplicate citations, non-peer-reviewed articles in which the abstract and full text were unavailable, and other languages.
Data extraction
After screening according to the three assessment steps for titles, abstracts, and full texts, the full text of each selected article was extracted for detailed analysis. The data were retrieved using a checklist recording author, publication year, type of study, mean age, sample size, number of positive tests, Effectiveness/Efficacy after one dose, Effectiveness/Efficacy after the second dose, and number of confirmed COVID cases, hospitalization, and death. From systematic search to final data extraction, all processes were followed independently by two research experts (PM, FM). After the screening, the data extraction was finally approved by (YM).
Risk of bias
The qualitative evaluation of studies was done according to the Newcastle-Ottawa Quality Assessment Scale (NOS) [ 25 ] by two of the authors (FM, YM). This scale is designed to evaluate the qualitative properties of observational studies (random clinical trials, case-control, retrospective, cohort, and cross-sectional studies). NOS examined each study through six items in three groups: selection, comparability, and exposure. Stars were given to each item, and the maximum score was 9. If the scores assigned to the published articles differed, the external discussion method would be used [ 26 , 27 ].
The Jadad checklist was used by two separate authors (PM and FM) to explore potential risks of bias in interventional studies. These scales include items to assess the adequacy of random sequence generation, allocation concealment, blinding, the detection of incomplete outcome data, selective outcome reporting, and other potential sources of bias [ 28 ].
Statistical analysis
The random-effects model was used to calculate the pooled Effectiveness/Efficacy with a 95% confidence interval (95% CI) with Metaprop order. Calculating the cumulative relative risk (RR) with the 95% confidence interval and the meta set command was used considering the relative risk’s logarithm and logarithm standard deviation. Statistical analysis was performed using STATA 16.0 (Stata Corp, College Station, TX, USA), and statistical significance was considered at P-Value < 0.05. Heterogeneity among studies was evaluated by applying the I square value and reported as a percentage (%) to show the extent of variation between studies. A forest plot was used for presenting the meta-analysis results schematically. Egger’s test and funnel plot were applied to evaluate the publication bias. In addition, a subgroup analysis was done to identify different sources of heterogeneity.
Results and discussion
Characteristics of included studies and the participants.
A total of 2622 publications were screened for evaluating two items about COVID-19 vaccines: (I) Efficacy and (II) Effectiveness. These two items were assessed according to the virus variant (Alpha, Beta, Delta, and Gamma) and the type of vaccine (AstraZeneca, Pfizer, Moderna, Janssen, and Bharat). Data on other vaccines were not included due to inadequate published data. Of these publications, 20 studies met the systematic reviews’ inclusion criteria (non-randomized and randomized) and were included in our meta-analysis (Fig. 1 ).
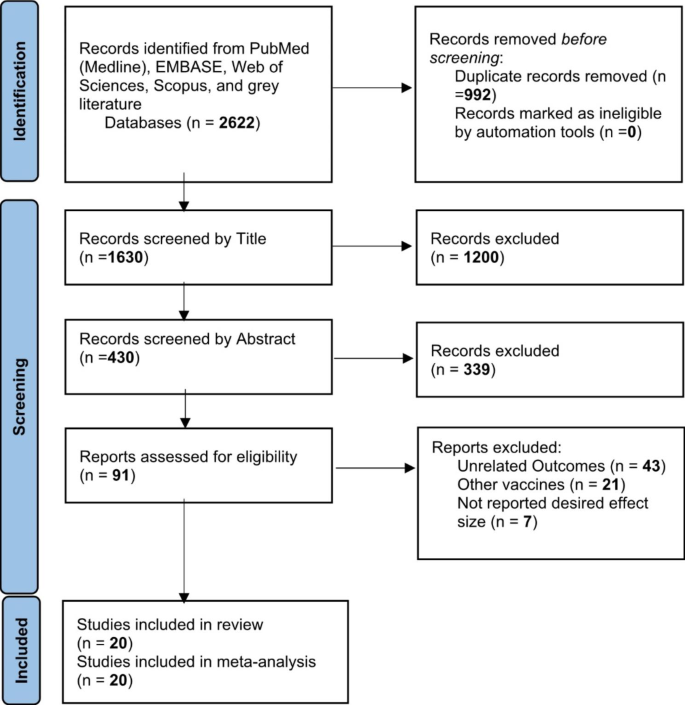
Identification of studies via databases and registers
One study was the cohort, four were randomized clinical trials (RCT), and fifteen were case-control. Clinical trials have evaluated vaccines’ efficacy, and observational studies such as cohorts and case controls have assessed their effectiveness. All selected papers were written in English. A total of 1,246,266 cases were included in this study that had received the COVID-19 vaccines. All vaccines were injected intramuscularly (IM). The participants were > 12 years old. The characteristics of included studies have been summarized in Table 1 .
The overall effectiveness of COVID-19 vaccines
After the first dose of the vaccine, the overall effectiveness of all COVID-19 vaccines was estimated to be 71% (95% CI 0.65, 0.78) (Fig. 2 ).
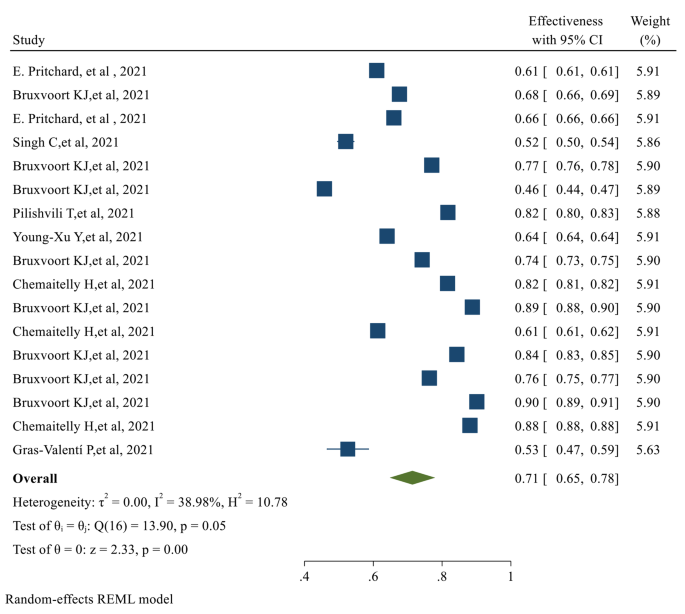
The overall Effectiveness of studied COVID-19 vaccines after the first dose
The overall Effectiveness of vaccines after the second dose was 91% (95% CI 0.88, 0.94), with a significant P-value ( p-value < 0.05 ) (Fig. 3 )
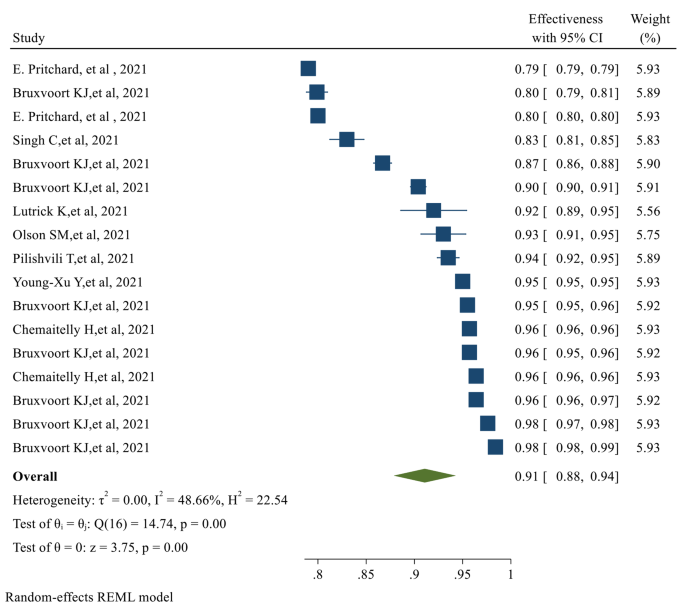
The overall Effectiveness of studied COVID-19 vaccines after the second dose. The overall Efficacy of COVID-19 vaccines
The overall Efficacy of the first dose of the vaccines evaluated in our study was 81% (95% CI 0.70, 0.91) (Fig. 4 )
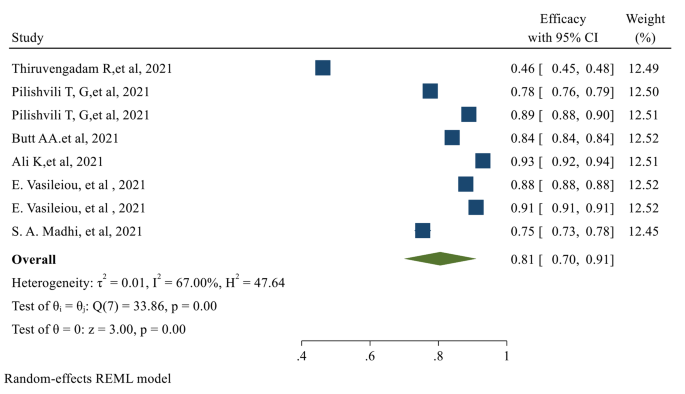
The overall Efficacy of the first dose of the studied vaccines
After the second dose of vaccination, the overall Efficacy of vaccines was 71% (95% CI 0.62, 0.79) with a significant P-value (Fig. 5 )
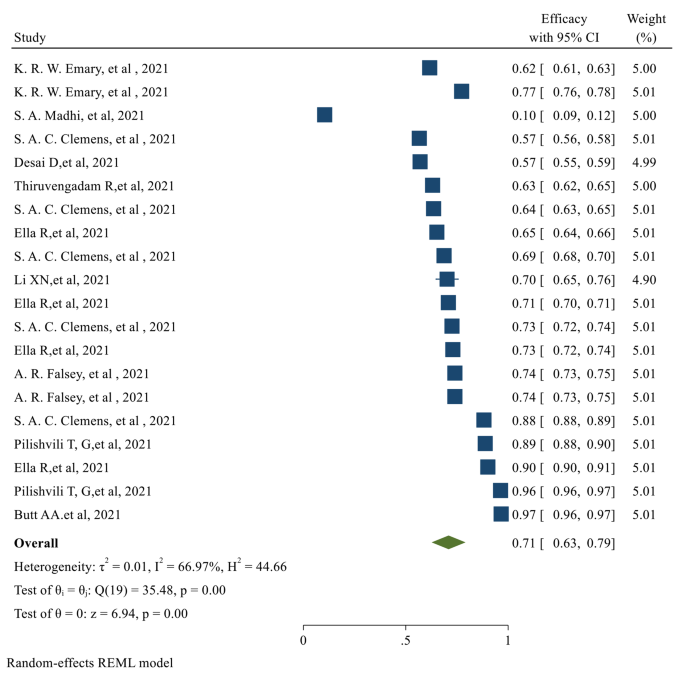
The overall Efficacy of the studied vaccines after the second dose
The individual efficacy of COVID-19 vaccines
The efficacy after the first dose was evaluated only in 8 of the selected studies, which assessed the efficacy of the AstraZeneca and Pfizer vaccines. No data was published on the efficacy after the first dose for Moderna, Johnson & Johnson, and Bharat. After the first dose of AstraZeneca and Pfizer vaccines, the pooled efficacy was 78% (95% CI 0.062, 0.95) and 84% (95% CI 0.77, 0.92), respectively. Of the selected publications, eighteen studies reported the efficacy after the second dose of vaccinations. The published data for the second dose Efficacy was only available for AstraZeneca, Pfizer, and Bharat vaccines. The second dose pooled Efficacy for AstraZeneca, Pfizer, and Bharat was 67% (95% CI 0.54, 0.80), 93% (95% CI 0.85, 1.00), and 71% (95% CI 0.61, 0.82) respectively (Table 2 ).
The individual effectiveness of COVID-19 vaccines
The first dose Effectiveness of the vaccines was evaluated in seventeen studies. For Moderna, AstraZeneca, and Pfizer, the pooled effectiveness after the first dose was 74% (95% CI 0.065, 0.83), 69% (95% CI 0.55, 0.82), and 67% (95% CI 0.51, 0.83) respectively. It was observed that the Effectiveness of Moderna after the first dose was higher than other types of vaccines. The second dose Effectiveness of the vaccines was reported in 17 studies. The pooled effectiveness after the second dose of Moderna, AstraZeneca, and Pfizer vaccines was 93% (95% CI 0.89, 0.97), 89% (0.80, 0.97), and 90% (95% CI 0.83, 0.96) respectively; Moderna had higher effectiveness after the second dose, among other studied vaccines (Table 2 ).
Efficacy of the vaccines against the virus variants
The overall first and second-dose vaccination Efficacy against different COVID-19 variants is listed in Table 2 . The first dose of overall vaccine Efficacy against the Alpha variant was 84%, which was higher than other variants (95% CI 0.84, 0.84). The overall efficacy of the first dose vaccination against the delta variant was only 46% (95% CI 0.45, 0.48), which was the lowest. Similarly, the highest second dose Efficacy was observed against the Alpha variant, which was 77% (95% CI 0.57, 0.97). The overall efficacy of the second dose against the Delta and Beta variants was 64% (95% CI 0.58, 0.69) and 10% (95% CI 0.09, 0.12), respectively.
Effectiveness of the vaccines against the virus variants
The overall first and second-dose vaccination Effectiveness against different COVID-19 variants is reported in Table 2 . The first dose Effectiveness of vaccination against the Gamma variant was 74% (95% CI 0.73, 0.75) which was more than other variants. However, the overall first dose Effectiveness was 82% (95% CI 0.81, 0.82). After the second dose, the highest effectiveness was against the Beta variant (96% (95% CI 0.96, 0.96)). The overall effectiveness after the second vaccination dose was 96% (95% CI 0.096, 0.96) (Table 2 ).
The risk of confirmed COVID infection after vaccination (risk ratio)
Two categories of the selected studies assessed the risk ratio of COVID after vaccination: observational and experimental. Only the pooled risk ratio of AstraZeneca was evaluated in the experimental studies, which was 50% (95% CI 0.35, 0.71). In the observational studies, AstraZeneca and Moderna had the lowest pooled risk ratios, which were 18% (95% CI 0.04, 0.84) and 19% (95% CI 0.17, 0.22), respectively. Bharat had the highest pooled risk ratio (82% (95% CI 0.75, 0.89) (Table 3 ); however, the number of studies on the Bharat vaccine was fewer than other types of vaccines. Based on the reported experimental studies for the vaccine variants, the Beta variant had the highest (79% (95% CI 0.43, 1.44)), and the Gamma variant had the lowest risk ratio (31% (95% CI 0.18, 0.54)). In the observational studies, Delta had the highest (52% (95% CI 0.27, 1.01), and Gamma had the lowest risk ratio (2% (95% CI 0.02, 0.02)) (Table 3 ).
Since the emergence of COVID-19, the effort to develop effective vaccines against the infection has been started. Due to the highly contagious nature of the virus, vaccination has been considered a significant measure in the fight against COVID-19. World Health Organization (WHO) allows countries to issue emergency use authorizations for COVID-19 vaccines in line with their national regulations and legislation. Domestic emergency use authorizations are issued at the countries’ discretion and are not subject to WHO approval. Up to now, several vaccines have been developed and marketed to limit the spread of COVID-19 infection. As of January 12, 2022, several COVID 19 vaccines have been given Emergency Use Listing (EUL), including those developed by Pfizer/BioNTech, AstraZeneca, Johnson & Johnson, Moderna, Sinopharm, Sinovac, Bharat Biotech, etc. [ 29 ].
Despite the significant role of COVID-19 vaccination in confining the infection, vaccines’ Efficacy and Effectiveness have not yet been comprehensively discussed. The present study meticulously looked into the Efficacy and Effectiveness of several vaccines.
Our analysis revealed that the overall effectiveness of the studied vaccines after the first dose is significantly less than their effectiveness after the second dose. The first dose’s effectiveness was evaluated in 17 studies. After the first dose, Moderna, AstraZeneca, and Pfizer’s Effectiveness was 74%, 69%, and 67%, respectively. The Effectiveness of Moderna after the first dose was higher than other types of studied vaccines. Second dose Effectiveness was evaluated in 17 studies. After the second dose of Moderna, AstraZeneca, and Pfizer vaccination, the effectiveness was 93%, 89%, and 90, respectively. Moderna provided higher effectiveness after the second dose among other studied vaccines. Therefore, administering the second dose should produce a more reliable response and higher effectiveness than a single dose.
Surprisingly, the overall efficacy of the first dose was significantly more than the second dose; 81% (95% CI 0.70, 0.91) for the first dose compared to 71% (95% CI 0.62, 0.79) for the second dose. This can be explained by the fact that the efficacy after the first dose was evaluated only in 8 studies that assessed only AstraZeneca and Pfizer vaccines. No data was available regarding the efficacy after the first dose of Moderna, Bharat, and Johnson & Johnson vaccines. We observed that the first dose Efficacy of the Pfizer vaccine is significantly more than the AstraZeneca vaccine. The Efficacy for AstraZeneca and Pfizer after the first dose vaccination was 78% and 84%, respectively. Concerning the second dose Efficacy, the published data were available only for AstraZeneca, Pfizer, and Bharat. In Total, eighteen studies evaluated the efficacy of these vaccines after the second dose. The Efficacy for AstraZeneca, Pfizer, and Bharat was 67%, 93%, and 71%, respectively.
We also investigated the Efficacy and Effectiveness of the first and second-dose vaccination against the COVID-19 virus variants. The overall efficacy of vaccination against the Alfa variant after the first dose was 84%, which was more than other variants. The highest efficacy after the second dose vaccination was also observed for the Alpha variant (77%). The first dose’s effectiveness against the Gamma variant was the highest (74%). Although, the overall first dose effectiveness was 82%. The highest second dose Effectiveness was against the Beta variant (96%), and the overall effectiveness after the second vaccination dose was 96% against all variants.
Up to now, there are other meta-analyses published on the efficacy and effectiveness of the COVID-19 vaccines. For example, in the meta-analysis reported by Pormohammad et., al, the efficacy of mRNA-based and adenovirus-vectored COVID-19 vaccines in phase II/III randomized clinical trial has been reported as 94.6% (95% CI 0.936–0.954) and 80.2% (95% CI 0.56–0.93), respectively. Additionally, the mRNA-based vaccines showed the highest reported side effects except for diarrhea and arthralgia [ 30 ]. However, the research had not reported the efficacy against different variants of the COVID-19 virus. Moreover, the Efficacy and Effectiveness of individual vaccines have not been mentioned; the vaccine Efficacy has been reported based on the vaccine classes. Another meta-analysis reported that the effectiveness of the Pfizer-BioNTech and Moderna vaccines was 91.2% and 98.1%, respectively, while the effectiveness of the CoronaVac vaccine was 65.7% in fully vaccinated individuals [ 31 ]. However, this study has not reported the effectiveness of the vaccines against COVID-19 variants or their efficacy.
Additionally, A previously reported network meta-analysis of various COVID-19 vaccines found Moderna was the most effective vaccine against COVID-19 infection, with an efficacy rate of 88%, followed by Sinopharm and Bharat. The least effective vaccines were Coronavac, Curevac, and AstraZeneca. The mRNA-based vaccines were superior in preventing infection and symptomatic infection, while the inactivated vaccines were most effective in preventing severe COVID-19 infection. Concerning safety, Sinopharm had the highest safety profile in local side effects, while ZF2001 had the highest safety in unsolicited side effects. Inactivated vaccines had the best safety profile in local and systemic side effects, while mRNA-based vaccines had the poorest safety profile. Thromboembolic events were reported after J&J, AstraZeneca, Pfizer, and Moderna vaccine administration. However, no confirmed vaccine-Induced Thrombotic Thrombocytopenia (VITT) cases were reported after mRNA vaccines [ 32 ].
It is necessary to mention that some vaccines’ overall or variant-specific Effectiveness and Efficacy are unavailable after the first or second dose. Moreover, the timing of the second dosing of the vaccines is not elicited in some trials, which may have led to the lower observed overall efficacy after the second dose. Additionally, some reports had noticeable bias by not including enough samples or not considering a broad enough geographical, economic, and age diversity.
We searched various databases and websites to include the maximum number of relevant publications to prevent database bias; after performing Egger’s regression test, we did not find significant publication bias. However, publication bias and heterogeneity for some pooled results must be considered when interpreting the outcomes.
Despite the valuable information provided by this meta-analysis, the study has some limitations to consider, such as the time frame of the studies (November 2020 to April 2022), the exclusion of unpublished data or ongoing investigations, the subjectivity of study selection criteria, and the limited number of vaccines evaluated. Additionally, the study did not consider differences in vaccine distribution among countries or provide data on the vaccines’ effectiveness against severe disease, hospitalization, or death. Despite its limitations, the meta-analysis highlights the need to continue monitoring the vaccines’ effectiveness.
In conclusion, Moderna, an mRNA-based vaccine, showed the highest total effectiveness after the first dose. Although the Pfizer vaccine showed a higher Efficacy after the first and second doses than AstraZeneca and Bharat, our conclusion has some limitations due to the lack of any published study regarding the Moderna and Johnson & Johnson vaccines’ efficacy. First-dose vaccination generally showed the highest overall effectiveness against the Gamma variant. Second dose vaccination showed a 96% overall Effectiveness against all variants. The efficacy of vaccination against the Alfa variant after the first dose was more than other variants. The highest efficacy after the second vaccination dose was also observed for the Alpha variant. Due to the timeline of the studies, all the vaccines are missing longer-term Efficacy and Effectiveness evaluations. This meta-analysis incorporated all relevant studies for summarizing and analyzing the Effectiveness and Efficacy of several vaccines for COVID-19. The results of this study support the overall Efficacy and Effectiveness of all studied COVID-19 vaccines and support the ongoing global public health effort for vaccination against COVID-19.
Data Availability
The data extracted for analyses are available by the corresponding author upon reasonable requests.
Abbreviations
Coronavirus Disease 2019
Severe Acute Respiratory Syndrome Coronavirus 2
World Health Organization
She J, et al. 2019 novel coronavirus of pneumonia in Wuhan, China: emerging attack and management strategies. Clin Transl Med. 2020;9(1):19.
Article PubMed PubMed Central Google Scholar
Lu R, et al. Genomic characterisation and epidemiology of 2019 novel coronavirus: implications for virus origins and receptor binding. Lancet. 2020;395(10224):565–74.
Article CAS PubMed PubMed Central Google Scholar
Zhu N, et al. A novel coronavirus from patients with Pneumonia in China, 2019. N Engl J Med. 2020;382(8):727–33.
Gilzad-Kohan H, Jamali F. Anti-inflammatory Properties of drugs used to Control COVID-19 and their Effects on the renin-angiotensin system and angiotensin-converting Enzyme-2. J Pharm Pharm Sci. 2020;23:259–77.
Article PubMed Google Scholar
Chen G, et al. Clinical and immunological features of severe and moderate coronavirus disease 2019. J Clin Invest. 2020;130(5):2620–9.
Guan WJ, et al. Clinical characteristics of Coronavirus Disease 2019 in China. N Engl J Med. 2020;382(18):1708–20.
Article CAS PubMed Google Scholar
Wang D, et al. Clinical characteristics of 138 hospitalized patients with 2019 Novel Coronavirus-Infected pneumonia in Wuhan, China. JAMA. 2020;323(11):1061–9.
Balasubramani J, Anbalagan M. Research productivity on COVID-19 in Dimension database: an analytical study. J Emerg Manag. 2021;18(7):63–9.
Chen T, et al. Clinical characteristics of 113 deceased patients with coronavirus disease 2019: retrospective study. BMJ. 2020;368:m1091.
Zhou F, et al. Clinical course and risk factors for mortality of adult inpatients with COVID-19 in Wuhan, China: a retrospective cohort study. Lancet. 2020;395(10229):1054–62.
Islam MI. Current drugs with potential for treatment of COVID-19: a Literature Review. J Pharm Pharm Sci. 2020;23(1):58–64.
Article Google Scholar
Çalık Başaran N, et al. Outcome of noncritical COVID-19 patients with early hospitalization and early antiviral treatment outside the ICU. Turk J Med Sci. 2021;51(2):411–20.
Tsang HF, et al. An update on COVID-19 pandemic: the epidemiology, pathogenesis, prevention and treatment strategies. Expert Rev Anti Infect Ther. 2021;19(7):877–88.
Singh AK, et al. Molnupiravir in COVID-19: a systematic review of literaturef. Diabetes Metab Syndr. 2021;15(6):102329.
WHO., WHO Coronavirus (COVID-19) Dashboard. 2021.
Checcucci E et al. The vaccine journey for COVID-19: a comprehensive systematic review of current clinical trials in humans. Panminerva Med, 2020.
Rego GNA et al. Current clinical trials protocols and the global effort for immunization against SARS-CoV-2. Vaccines (Basel), 2020. 8(3).
Smit M, et al. Prophylaxis for COVID-19: a systematic review. Clin Microbiol Infect. 2021;27(4):532–7.
WHO., The COVID-19 vaccine tracker. 2021.
Francis AI, et al. Review of COVID-19 vaccine subtypes, efficacy and geographical distributions. Postgrad Med J. 2022;98(1159):389–94.
Polack FP, et al. Safety and efficacy of the BNT162b2 mRNA Covid-19 vaccine. New England Journal of Medicine; 2020.
Sadoff J, et al. Safety and efficacy of single-dose Ad26. COV2. S vaccine against Covid-19. N Engl J Med. 2021;384(23):2187–201.
Kumar VM, et al. Strategy for COVID-19 vaccination in India: the country with the second highest population and number of cases. npj Vaccines. 2021;6(1):1–7.
Moher D, et al. Preferred reporting items for systematic reviews and meta-analyses: the PRISMA statement. Ann Intern Med. 2009;151(4):264–9.
Wells G et al. The Newcastle-Ottawa Quality Assessment Scale (NOS) for assessing the quality of non-randomized studies in meta-analyses. Clin Epidemiol [Internet], 2017: p. 1–2.
Von Elm E, et al. The strengthening the reporting of Observational Studies in Epidemiology (STROBE) statement: guidelines for reporting observational studies. Ann Intern Med. 2007;147(8):573–7.
Von Elm E, et al. The strengthening the reporting of Observational Studies in Epidemiology (STROBE) statement: guidelines for reporting observational studies. J Clin Epidemiol. 2008;61(4):344–9.
Moher D, et al. Assessing the quality of randomized controlled trials: an annotated bibliography of scales and checklists. Control Clin Trials. 1995;16(1):62–73.
WHO. Coronavirus disease (COVID-19): Vaccines. 2022; Available from: https://www.who.int/news-room/questions-and-answers/item/coronavirus-disease-(covid-19)-vaccines .
Pormohammad A et al. Efficacy and safety of COVID-19 vaccines: a systematic review and Meta-analysis of Randomized clinical trials. Vaccines (Basel), 2021. 9(5).
Zheng C, et al. Real-world effectiveness of COVID-19 vaccines: a literature review and meta-analysis. Int J Infect Dis. 2022;114:252–60.
Toubasi AA, et al. Efficacy and safety of COVID-19 vaccines: a network meta-analysis. J Evid Based Med. 2022;15(3):245–62.
Download references
Acknowledgements
Not applicable.
Author information
Authors and affiliations.
Department of Pharmaceutical and Administrative Sciences, College of Pharmacy, Western New England University, 1215 Wilbraham Road, Springfield, MA, 01119, USA
Marzieh Soheili
Department of Physical Medicine and Rehabilitation, School of Medicine, Hamedan University of Medical Sciences, Hamedan, Iran
Sorour Khateri
Social Determinants of Health Research Center, Research Institute for Health Development, Kurdistan University of Medical Sciences, Sanandaj, Iran
Farhad Moradpour & Yousef Moradi
Department of Epidemiology and Biostatistics, School of Medicine, Kurdistan University of Medical Sciences, Sanandaj, Iran
Pardis Mohammadzedeh & Mostafa Zareie
Pediatric Gastroenterology Fellowship, Department of Pediatrics, School of Medicine, Namazi teaching Hospital, Shiraz University of Medical Sciences, Shiraz, Iran
Seyede Maryam Mahdavi Mortazavi
Massachusetts College of Pharmacy and Health Sciences (MCPHS), 179 Longwood Avenue, Boston, MA, 02115, USA
Sima Manifar
Hamed Gilzad Kohan
You can also search for this author in PubMed Google Scholar
Contributions
Study concept and design: YM. Acquisition, analysis, and interpretation of data: YM, MS, HGK, FM, PM, SK, MZ, SM, and SMMM. Drafting of the manuscript: YM, HGK, MS. Critical revision of the manuscript for the important intellectual content: YM, MS. Project administration: YM and HGK. All authors have approved the final manuscript draft.
Corresponding authors
Correspondence to Hamed Gilzad Kohan or Yousef Moradi .
Ethics declarations
Ethics approval and consent to participate, consent for publication, competing interests.
The authors declare no competing interests.
Additional information
Publisher’s note.
Springer Nature remains neutral with regard to jurisdictional claims in published maps and institutional affiliations.
Rights and permissions
Open Access This article is licensed under a Creative Commons Attribution 4.0 International License, which permits use, sharing, adaptation, distribution and reproduction in any medium or format, as long as you give appropriate credit to the original author(s) and the source, provide a link to the Creative Commons licence, and indicate if changes were made. The images or other third party material in this article are included in the article’s Creative Commons licence, unless indicated otherwise in a credit line to the material. If material is not included in the article’s Creative Commons licence and your intended use is not permitted by statutory regulation or exceeds the permitted use, you will need to obtain permission directly from the copyright holder. To view a copy of this licence, visit http://creativecommons.org/licenses/by/4.0/ . The Creative Commons Public Domain Dedication waiver ( http://creativecommons.org/publicdomain/zero/1.0/ ) applies to the data made available in this article, unless otherwise stated in a credit line to the data.
Reprints and permissions
About this article
Cite this article.
Soheili, M., Khateri, S., Moradpour, F. et al. The efficacy and effectiveness of COVID-19 vaccines around the world: a mini-review and meta-analysis. Ann Clin Microbiol Antimicrob 22 , 42 (2023). https://doi.org/10.1186/s12941-023-00594-y
Download citation
Received : 04 October 2022
Accepted : 10 May 2023
Published : 19 May 2023
DOI : https://doi.org/10.1186/s12941-023-00594-y
Share this article
Anyone you share the following link with will be able to read this content:
Sorry, a shareable link is not currently available for this article.
Provided by the Springer Nature SharedIt content-sharing initiative
- Effectiveness
Annals of Clinical Microbiology and Antimicrobials
ISSN: 1476-0711
- Submission enquiries: [email protected]
Thank you for visiting nature.com. You are using a browser version with limited support for CSS. To obtain the best experience, we recommend you use a more up to date browser (or turn off compatibility mode in Internet Explorer). In the meantime, to ensure continued support, we are displaying the site without styles and JavaScript.
- View all journals
- Explore content
- About the journal
- Publish with us
- Sign up for alerts
- Review Article
- Published: 12 April 2021

Vaccine development for emerging infectious diseases
- Jean-Louis Excler ORCID: orcid.org/0000-0002-6462-5101 1 ,
- Melanie Saville 2 ,
- Seth Berkley 3 &
- Jerome H. Kim ORCID: orcid.org/0000-0003-0461-6438 1
Nature Medicine volume 27 , pages 591–600 ( 2021 ) Cite this article
76k Accesses
238 Citations
93 Altmetric
Metrics details
- Viral infection
Examination of the vaccine strategies and technical platforms used for the COVID-19 pandemic in the context of those used for previous emerging and reemerging infectious diseases and pandemics may offer some mutually beneficial lessons. The unprecedented scale and rapidity of dissemination of recent emerging infectious diseases pose new challenges for vaccine developers, regulators, health authorities and political constituencies. Vaccine manufacturing and distribution are complex and challenging. While speed is essential, clinical development to emergency use authorization and licensure, pharmacovigilance of vaccine safety and surveillance of virus variants are also critical. Access to vaccines and vaccination needs to be prioritized in low- and middle-income countries. The combination of these factors will weigh heavily on the ultimate success of efforts to bring the current and any future emerging infectious disease pandemics to a close.
Similar content being viewed by others
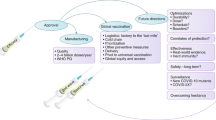
Looking beyond COVID-19 vaccine phase 3 trials
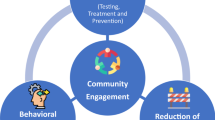
Leveraging lessons learned from the COVID-19 pandemic for HIV
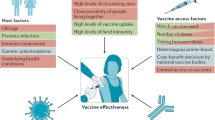
Progress of the COVID-19 vaccine effort: viruses, vaccines and variants versus efficacy, effectiveness and escape
Newly emerging and reemerging infectious viral diseases have threatened humanity throughout history. Several interlaced and synergistic factors including demographic trends and high-density urbanization, modernization favoring high mobility of people by all modes of transportation, large gatherings, altered human behaviors, environmental changes with modification of ecosystems and inadequate global public health mechanisms have accelerated both the emergence and spread of animal viruses as existential human threats. In 1918, at the time of the ‘Spanish flu’, the world population was estimated at 1.8 billion. It is projected to reach 9.9 billion by 2050, an increase of more than 25% from the current 2020 population of 7.8 billion ( https://www.worldometers.info ). The novel severe acute respiratory syndrome coronavirus 2 (SARS-CoV-2) responsible for the coronavirus disease 2019 (COVID-19) pandemic 1 , 2 , 3 engulfed the entire world in less than 6 months, with high mortality in the elderly and those with associated comorbidities. The pandemic has severely disrupted the world economy. Short of lockdowns, the only means of control have been limited to series of mitigation measures such as self-distancing, wearing masks, travel restrictions and avoiding gatherings, all imperfect and constraining. Now with more than 100 million people infected and more than 2 million deaths, it seems that the addition of vaccine(s) to existing countermeasures holds the best hope for pandemic control. Taken together, these reasons compel researchers and policymakers to be vigilant, reexamine the approach to surveillance and management of emerging infectious disease threats, and revisit global mechanisms for the control of pandemic disease 4 , 5 .
Emerging and reemerging infectious diseases
The appearance of new infectious diseases has been recognized for millennia, well before the discovery of causative infectious agents. Despite advances in development of countermeasures (diagnostics, therapeutics and vaccines), world travel and increased global interdependence have added layers of complexity to containing these infectious diseases. Emerging infectious diseases (EIDs) are threats to human health and global stability 6 , 7 . A review of emerging pandemic diseases throughout history offers a perspective on the emergence and characteristics of coronavirus epidemics, with emphasis on the SARS-CoV-2 pandemic 8 , 9 . As human societies grow in size and complexity, an endless variety of opportunities is created for infectious agents to emerge into the unfilled ecologic niches we continue to create. To illustrate this constant vulnerability of populations to emerging and reemerging pathogens and their respective risks to rapidly evolve into devastating outbreaks and pandemics, a partial list of emerging viral infectious diseases that occurred between 1900 and 2020 is shown in Table 1 .
Although nonemerging infectious diseases (not listed in Table 1 ), two other major mosquito-borne viral infections are yellow fever and dengue. Yellow fever, known for centuries and an Aedes mosquito-borne disease, is endemic in more than 40 countries across Africa and South America. Since 2016, several yellow fever outbreaks have occurred in Angola, Democratic Republic of Congo, Nigeria and Brazil to cite a few 10 , raising major concerns about the adequacy of yellow fever vaccine supply. Four live attenuated vaccines derived from the live attenuated yellow fever strain (17D) 11 and prequalified by the WHO (World Health Organization) are available 12 .
Dengue is an increasing global public health threat with the four dengue virus types (DENV1–4) now cocirculating in most dengue endemic areas. Population growth, an expansion of areas hospitable for Aedes mosquito species and the ease of travel have all contributed to a steady rise in dengue infections and disease. Dengue is common in more than 100 countries around the world. Each year, up to 400 million people acquire dengue. Approximately 100 million people get sick from infection, and 22,000 die from severe dengue. Most seriously affected by outbreaks are the Americas, South/Southeast Asia and the Western Pacific; Asia represents ~70% of the global burden of disease ( https://www.cdc.gov/dengue ). Several vaccines have been developed 13 . A single dengue vaccine, Sanofi Pasteur’s Dengvaxia based on the yellow fever 17D backbone, has been licensed in 20 countries, but uptake has been poor. A safety signal in dengue-seronegative vaccine recipients stimulated an international review of the vaccine performance profile, new WHO recommendations for use and controversy in the Philippines involving the government, regulatory agencies, Sanofi Pasteur, clinicians responsible for testing and administering the vaccine, and the parents of vaccinated children 14 .
Two bacterial diseases, old scourges of humanity, are endemic and responsible for recurrent outbreaks and are increasingly antimicrobial resistant. Cholera, caused by pathogenic strains of Vibrio cholerae , is currently in its seventh global pandemic since 1817; notably, the seventh pandemic started in 1961 15 . Global mortality due to cholera infection remains high, mainly due to delay in rehydrating patients. The global burden of cholera is estimated to be between 1.4 and 4.3 million cases with about 21,000–143,000 deaths per year, mostly in Asia and Africa. Tragic outbreaks have occurred in Yemen and Haiti. Adding to rehydration therapy, antibiotics have been used in the treatment of cholera to shorten the duration of diarrhea and to limit bacterial spread. Over the years, antimicrobial resistance developed in Asia and Africa to many useful antibiotics including chloramphenicol, furazolidone, trimethoprim-sulfamethoxazole, nalidixic acid, tetracycline and fluoroquinolones. Several vaccines have been developed and WHO prequalified; these vaccines constitute a Gavi-supported global stockpile for rapid deployment during outbreaks 16 .
Typhoid fever is a severe disease caused by the Gram-negative bacterium Salmonella enterica subsp. enterica serovar Typhi ( S . Typhi). Antimicrobial-resistant S . Typhi strains have become increasingly common. The first large-scale emergence and spread of a novel extensively drug-resistant (XDR) S . Typhi clone was first reported in Sindh, Pakistan 17 , 18 , and has subsequently been reported in India, Bangladesh, Nepal, the Philippines, Iraq and Guatemala 19 , 20 . The world is in a critical period as XDR S . Typhi has appeared in densely populated areas. The successful development of improved typhoid vaccines (conjugation of the Vi polysaccharide with a carrier protein) with increased immunogenicity and efficacy including in children less than 2 years of age will facilitate the control of typhoid, in particular in XDR areas by decreasing the incidence of typhoid fever cases needing antibiotic treatment 21 , 22 .
A model of vaccine development for emerging infectious diseases
The understanding of emerging infectious diseases has evolved over the past two decades. A look back at the SARS-CoV outbreak in 2002 shows that—despite a small number of deaths and infections—its high mortality and transmissibility caused significant global disruption (see Table 1 ). The epidemic ended as work on vaccines was initiated. Since then, the disease has not reappeared—wet markets were closed and transmission to humans from civets ceased. Consequently, work on vaccines against SARS-CoV ended and its funding was cut. Only a whole inactivated vaccine 23 and a DNA vaccine 24 were tested in phase 1 clinical trials.
Following a traditional research and development pipeline, it takes between 5 and 10 years to develop a vaccine for an infectious agent. This approach is not well suited for the needs imposed by the emergence of a new pathogen during an epidemic. Figure 1 shows a comparison of the epidemic curves and vaccine development timelines between the 2014 West African Ebola outbreak and COVID-19. The 2014 Ebola epidemic lasted more than 24 months with 11,325 deaths and was sufficiently prolonged to enable the development and testing of vaccines for Ebola, with efficacy being shown for one vaccine (of several) toward the end of the epidemic 25 , 26 . What makes the COVID-19 pandemic remarkable is that the whole research and development pipeline, from the first SARS-CoV-2 viral sequenced to interim analyses of vaccine efficacy trials, was accomplished in just under 300 days 27 . Amid increasing concerns about unmitigated transmission during the 2013–2016 Western African Ebola outbreak in mid-2014, WHO urged acceleration of the development and evaluation of candidate vaccines 25 . To ensure that manufacturers would take the Ebola vaccine to full development and deployment, Gavi, the Vaccine Alliance, publicly announced support of up to US$300 million for vaccine purchase and followed that announcement with an advance purchase agreement. Ironically, there had been Ebola vaccines previously developed and tested for biodefense purposes in nonhuman primates, but this previous work was neither ‘ready’ for clinical trials during the epidemic nor considered commercially attractive enough to finish development 28 .
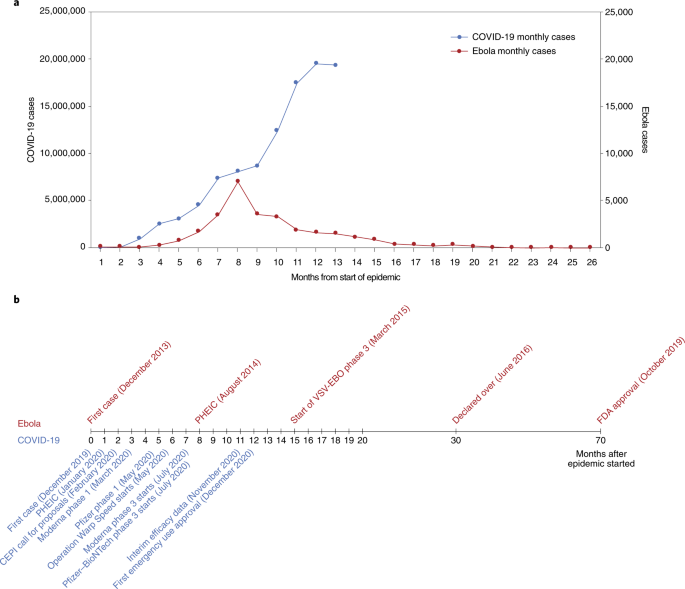
a , The number of months from the onset of the epidemic is shown against the number of reported cases per day. Note that the COVID-19 (left) and Ebola (right) axes are scaled differently. b , Vaccine development timelines for COVID-19 versus Ebola in the context of particular events during the respective outbreaks. PHEIC, public health emergency of international concern.
From these perceived shortcomings in vaccine development during public health emergencies arose the Coalition for Epidemic Preparedness Innovations (CEPI), a not-for-profit organization dedicated to timely vaccine development capabilities in anticipation of epidemics 29 , 30 . CEPI initially focused on diseases chosen from a list of WHO priority pathogens for EIDs—Middle East respiratory syndrome (MERS), Lassa fever, Nipah, Rift Valley fever (RVF) and chikungunya. The goal of CEPI was to advance candidate vaccines through phase 2 and to prepare stockpiles of vaccine against eventual use/testing under epidemic circumstances. CEPI had also prepared for ‘disease X’ by investing in innovative rapid response platforms that could move from sequence to clinical trials in weeks rather than months or years, such as mRNA and DNA technology, platforms that were useful when COVID-19 was declared a global health emergency in January 2020, and a pandemic in March 2020 31 , 32 .
CEPI has been able to fund several vaccine development efforts, among them product development by Moderna, Inovio, Oxford–AstraZeneca and Novavax. Providing upfront funding helped these groups to advance vaccine candidates to clinical trials and develop scaled manufacturing processes in parallel, minimizing financial risk to vaccine developers. The launch of the larger US-funded Operation Warp Speed 33 further provided companies with funding—reducing risks associated with rapid vaccine development and securing initial commitments in vaccine doses.
Vaccine platforms and vaccines for emerging infectious diseases
Vaccines are the cornerstone of the management of infectious disease outbreaks and are the surest means to defuse pandemic and epidemic risk. The faster a vaccine is deployed, the faster an outbreak can be controlled. As discussed in the previous section, the standard vaccine development cycle is not suited to the needs of explosive pandemics. New vaccine platform technologies however may shorten that cycle and make it possible for multiple vaccines to be more rapidly developed, tested and produced 34 . Table 2 provides examples of the most important technical vaccine platforms for vaccines developed or under development for emerging viral infectious diseases. Two COVID-19 vaccines were developed using mRNA technology (Pfizer–BioNTech 35 and Moderna 36 ), both showing safety and high efficacy, and now with US Food and Drug Administration (FDA) emergency use authorization (EUA) 37 , 38 and European Medicines Agency (EMA) conditional marketing authorization 39 , 40 . While innovative and encouraging for other EIDs, it is too early to assert that mRNA vaccines represent a universal vaccine approach that could be broadly applied to other EIDs (such as bacterial or enteric pathogens). While COVID-19 mRNA vaccines are a useful proof of concept, gathering lessons from their large-scale deployment and effectiveness studies still requires more work and time.
While several DNA vaccines are licensed for veterinary applications, and DNA vaccines have shown safety and immunogenicity in human clinical trials, no DNA vaccine has reached licensure for use in humans 41 . Recombinant proteins vary greatly in design for the same pathogen (for example, subunit, virus-like particles) and are often formulated with adjuvants but have longer development times. Virus-like particle-based vaccines used for hepatitis B and human papillomavirus are safe, highly immunogenic, efficacious and easy to manufacture in large quantity. The technology is also easily transferable. Whole inactivated pathogens (for example, SARS-CoV-2, polio, cholera) or live attenuated vaccines (for example, SARS-CoV-2, polio, chikungunya) are unique to each pathogen. Depending on the pathogen, these vaccines also may require biosafety level 3 manufacturing (at least for COVID-19 and polio), which may limit the possibility of technology transfer for increasing the global manufacturing capacity.
Other vaccines are based on recombinant vector platforms, subdivided into nonreplicating vectors (for example, adenovirus 5 (Ad5), Ad26, chimpanzee adenovirus-derived ChAdOx, highly attenuated vectors like modified vaccinia Ankara (MVA)) and live attenuated vectors such as the measles-based vector or the vesicular stomatitis virus (VSV) vector. Either each vector is designed with specific inserts for the pathogen targeted, or the same vector can be designed with different inserts for the same disease. The development of the Merck Ebola vaccine is an example. ERVEBO is a live attenuated, recombinant VSV-based, chimeric-vector vaccine, where the VSV envelope G protein was deleted and replaced by the envelope glycoprotein of Zaire ebolavirus . ERVEBO is safe and highly efficacious, now approved by the US FDA and the EMA, and WHO prequalified, making VSV an attractive ‘platform’ for COVID-19 and perhaps for other EID vaccines 26 although the −70 °C ultracold chain storage requirement still presents a challenge.
Other equally important considerations are speed of development, ease of manufacture and scale-up, ease of logistics (presentation, storage conditions and administration), technology transfer to other manufacturers to ensure worldwide supply, and cost of goods. Viral vectors such as Ad5, Ad26 and MVA have been used in HIV as well as in Ebola vaccines 42 . Finally, regulatory authorities do not approve platforms but vaccines. Each vaccine is different. However, with each use of a specific technology, regulatory agencies may, over time, become more comfortable with underlying technology and the overall safety and efficacy of the vaccine platform, allowing expedited review and approvals in the context of a pandemic 43 . With COVID-19, it meant that the regulatory authorities could permit expedited review of ‘platform’ technologies, such as RNA and DNA, that had been used (for other conditions) and had safety profiles in hundreds of people.
A heterologous prime–boost (HPB) vaccine approach has been extensively explored for HIV 44 and Ebola vaccines 42 . It is being investigated for COVID-19 vaccines with the Oxford–AstraZeneca AZD1222 and Gamaleya Sputnik V COVID-19 vaccines 45 or with the Pfizer–BioNTech vaccine ( https://www.comcovstudy.org.uk ). Other HPB combinations might be considered involving mRNA, DNA, viral vector-based and protein-based vaccines. This may offer the potential benefit of improving the immune response and avoiding mutlidose reactogenicity or anti-vector immune responses. Additionally, people previously vaccinated with the standard regimen (for example, single or two dose) could be offered a booster immunization with a different vaccine. This might mitigate current shortages in vaccines, particularly in low- and middle-income countries (LMICs). Such a matrix of HPB possibilities deserves further consideration by manufacturers, funders and regulators supported by clinical trial studies and assessment of implementation challenges.
Important improvements could speed up availability. Standardized labeling of vaccines so that they can be interchanged across countries and regions, date of production rather than expiration so that shelf life can be tracked, three-dimensional bar coding to allow critical information to be updated, standard indemnification and liability language that would allow agreement with all manufacturers, a no-fault compensation mechanism for serious adverse events related to vaccine administration, and regulatory harmonization are all critical and being worked on as part of the COVID-19 vaccine response and must be optimized for future outbreaks.
The pathway to EUA, licensure and beyond
Big pharmaceutical or biotechnology companies supported by organizations such as CEPI or efforts such as Operation Warp Speed have conducted efficacy trials in countries or regions with the highest SARS-CoV-2 incidence rates. The same groups have also committed funding for large-scale manufacturing at risk. With more than 60 vaccine candidates in clinical trials and another 170 in preclinical development (WHO COVID-19 vaccine landscape) 46 , it is uncertain whether vaccine candidates not in the first wave of testing/approvals will be able to progress to EUA and licensure based solely on results of randomized clinical efficacy trials with clinical endpoints. Regulators and ethics committees may decide that noninferiority clinical trials against comparator vaccines with proven clinical efficacy will be needed for further approvals. Would the demonstration of equivalence between immune responses generated by a new vaccine and those of a clinically proven efficacious vaccine (bridging studies) 47 be accepted by regulatory authorities and replace the need for noninferiority clinical endpoint studies? For that to occur there must be agreement on what are immune correlates of protection (ICP) to COVID-19, and these have yet to be identified. Moreover, it is not yet clear that ICP will translate equally between different vaccine platforms; for example, are immune responses generated by chimpanzee adenovirus the same as those generated by proteins or whole inactivated virus? As incidence rates of a disease decrease over time due to sustained mitigation measures and implementation of vaccination, larger sample sizes in multicountry trials, additional participant accrual time and complex logistics will likely be required for future approvals, compromising the speed of clinical development and increasing cost. Early deployment of scarce doses of still-investigational vaccines (under emergency use listing (EUL) or similar regulatory mechanisms) could bring additional public health benefits if accompanied by firm commitment to maintaining blinded follow-up of participants in ongoing or future placebo-controlled trials until a licensed vaccine is fully deployed in the population 48 .
Randomized controlled trials might underestimate the protective effect of vaccines at the population level. This would occur if the COVID-19 vaccine, in addition to conferring direct protection to individuals, reduces transmission of COVID-19 between individuals, providing protection to unvaccinated individuals and enhanced protection of vaccinated individuals in contact with vaccinated individuals. Vaccine-induced herd protection, which might be crucial to the public health value of a vaccine, will be missed when trials are individually randomized and analyses fail to take account of the geographical distribution of individuals in the population 49 . For these reasons, other clinical trial designs have been proposed once COVID-19 vaccines have achieved licensure via current phase 3 trials to assess how useful the vaccines will be in practice and addressing vaccine effectiveness, including the level of protection of both vaccinated and nonvaccinated individuals in targeted populations 50 .
In the particular context of the COVID-19 pandemic, whether regulatory authorities would require clinical endpoints in future efficacy trials or would consider ICP remains unclear. Clinical endpoints provide increased accuracy with regard to definitive clinical outcomes where outcome-related analyses using ICP are inferential. ICP will contribute to our understanding of viral pathogenesis and immunity, be useful for future approval of vaccines, and help in our understanding of waning of protective immunity following vaccination or infection. The paradox is that the higher the efficacy, the more difficult it will be to identify these correlates because there may not be enough infected vaccine recipients to compare with uninfected vaccine recipients. The analysis of ICP may be possible only in clinical trials showing a lower vaccine efficacy 50 . They would also not provide a rigorous evaluation of long-term safety and the potential for vaccine-associated enhanced respiratory disease 51 .
Pharmacovigilance and surveillance
In May 2020, the 42nd Global Advisory Committee on Vaccine Safety addressed pharmacovigilance preparedness for the launch of the future COVID-19 vaccines 52 . One of their recommendations was that infrastructure and capacity for surveillance of the safety of COVID-19 vaccines should be in place in all countries and engaged before a vaccine is introduced. The WHO’s COVID-19 vaccine safety surveillance manual develops the monitoring and reporting of adverse events following immunization and adverse events of special interest, data management systems and safety communication, and the need for postauthorization safety surveillance studies 53 . One critical element of this surveillance is the duration of the observation period. The implementation of this surveillance will require local, national, regional and global collaboration. While countries should include preparedness plans for COVID-19 vaccine safety in their overall plans for vaccine introduction, building on WHO guidance, it is imperative that the COVID-19 Vaccines Global Access (COVAX) initiative (coordinated by CEPI, Gavi, the Vaccine Alliance, and WHO) works with partners on capacity building and the practical aspects of implementation with technical and training support tailored to the settings.
In view of the public health urgency and the extensive vaccination campaigns foreseen worldwide, the EMA and the national competent authorities in EU member states have prepared themselves for the expected high data volume by putting pharmacovigilance plans specific for COVID-19 vaccines in place. Good pharmacovigilance practices include detailed requirements and guidance on the principles of a risk management plan (RMP) and requirements for vaccines. In addition, core RMP requirements for COVID-19 vaccines have been developed to facilitate and harmonize the preparation of RMPs by companies and their evaluation by assessors. The RMP preparation addresses the planning of the postauthorization safety follow-up of COVID-19 vaccines by marketing authorization holders, while acknowledging uncertainties in the pandemic setting and recommending ways to prepare for pharmacovigilance activities 54 . Similarly, the US Advisory Committee on Immunization Practices (ACIP) initially convened the COVID-19 Vaccine Safety Technical Working Group in June 2020 to advise the ACIP COVID-19 Vaccine Workgroup and the full ACIP on the safety monitoring of COVID-19 vaccines under development and pharmacovigilance postapproval 55 .
Key lessons could be learnt from past situations where new vaccines were introduced in response to pandemic and epidemic emergencies. For the 2009 H1N1 influenza pandemic, few countries had a pandemic preparedness plan that comprehensively addressed vaccine deployment and monitoring of adverse events. The African Vaccine Regulatory Forum, a regional network of regulators and ethics committees, working closely with regulators from other parts of the world, participated in the review of clinical trial protocols and results, the joint monitoring of trials and the joint authorization and deployment of vaccines 56 . Such models can be used to guide pharmacovigilance for the deployment of COVID-19 vaccines, particularly in LMICs with limited resources. The introduction of the first licensed dengue vaccine, while not in the context of an international public health emergency, illustrated a number of lessons for the pharmacovigilance of newly introduced vaccines, particularly the vaccine-associated enhanced disease that was observed 13 , 14 . Due to the significant sequence homology between SARS-CoV-2 and SARS-CoV, antibody-dependent enhancement (ADE) and vaccine-associated enhanced respiratory disease (VAERD) were raised as potential safety issues 57 , 58 . VAERD and ADE have not been described in current reports of SARS-CoV-2 vaccine phase 3 trials. Similarly, VAERD has not been reported in animal challenge studies with SARS-CoV-2 vaccines that conferred protection 50 . With ADE the effect of waning antibody titers after vaccination (or after infection) and potential safety signals are unknown, which emphasizes the importance of follow-up monitoring 57 .
Pregnant women seem to be disproportionately affected during pandemics and emerging pathogen outbreaks 59 , 60 . The Pregnancy Research Ethics for Vaccines, Epidemics, and New Technologies (PREVENT) Working Group has published a roadmap to guide the inclusion of the interests of pregnant women in the development and deployment of vaccines against emerging pathogens 61 , 62 .
Equally important is the surveillance on SARS-CoV-2 circulating strains as well as of other coronaviruses (MERS, seasonal) 63 . SARS-CoV-2 is evolving, with new lineages being reported all over the world. Amongst previous lineages, D614G was shown to have faster growth in vitro and enhanced transmission in small animals, and has subsequently become globally dominant 64 , 65 , 66 . Other variants of concern have been described in the UK (B.1.1.7) 67 and in Brazil (B.1.1.28.1/P1) 68 with higher capacity for transmission and, potentially, lethality. N501Y (B.1.351) isolated in South Africa has an increased affinity for the human ACE2 receptor, which together with the repeated and independent evolution of 501Y-containing lineages 69 strongly argues for enhanced transmissibility. The B.1.351 variant has nine spike alterations; it rapidly emerged in South Africa during the second half of 2020 and has shown resistance to neutralizing antibodies elicited by infection and vaccination with previously circulating lineages. The AstraZeneca COVID-19 vaccine rollout in South Africa was recently halted after the analysis showed minimal efficacy against mild and moderate cases due to B.1.351, which accounts for 90% of the cases in this country 70 . The Novavax vaccine efficacy is 86% against the variant identified in the UK and 60% against the variant identified in South Africa 71 . The efficacy of a single dose of Johnson & Johnson’s Ad26 was 57% against moderate to severe COVID-19 infection in South Africa 72 .
For the many people who have already been infected with SARS-CoV-2 globally and are presumed to have accumulated some level of immunity, new variants such as B.1.351 pose a significant reinfection risk, although vaccine-induced cell-mediated immune responses might mitigate this risk. Scientists do not know how variant lineages will evolve under vaccine-induced immune pressure during the vaccination rollout or whether choices that alter the schedule or dose may impact virus evolution. Whether vaccines efficacious against current circulating strains including the variants identified in the UK and Brazil will keep their efficacy against emerging variants is unknown and deserves enhanced global COVID-19 surveillance in both humans and animals, similar to those developed for influenza. Global influenza surveillance has been conducted through WHO’s Global Influenza Surveillance and Response System since 1952. The Global Influenza Surveillance and Response System is a global mechanism of surveillance, preparedness and response for seasonal, pandemic and zoonotic influenza, a global platform for monitoring influenza epidemiology and disease, and a global alert system for novel influenza viruses and other respiratory pathogens 73 . The Global Initiative on Sharing Avian Influenza Database ( https://www.gisaid.org ) promotes the rapid sharing of data from all influenza viruses and the coronavirus causing COVID-19. These include genetic sequence and related clinical and epidemiological data associated with human viruses, and geographical as well as species-specific data associated with avian and other animal viruses. This molecular epidemiology surveillance should be expanded to all EIDs, particularly the deadliest and most transmissible, as recently described for Ebola 25 . As with influenza, preparations for SARS-CoV-2 vaccine variants should be proactive, with a view that platforms such as mRNA could generate new vaccine strains very rapidly. A clear regulatory pathway for strain change needs discussion with the regulators.
Approval process for licensure and EUA and the risk of speed
Vaccines are classically approved by the country’s national regulatory authority such as the US FDA or by a centralized procedure through the EMA. Once approved for licensure by a stringent or functional national regulatory authority in the country of manufacture, the manufacturing company can submit a dossier for WHO prequalification. However, for SARS-CoV-2 vaccines intended for COVAX, WHO prequalification is not required for initial use if they have received WHO EUL. COVAX is one of three pillars of the Access to COVID-19 Tools Accelerator, which was launched in April 2020 by the WHO, the EC (European Commission) and France. Vaccines receiving WHO EUL can be purchased by UNICEF (United Nations International Children’s Emergency Fund), the largest purchaser of vaccines for Gavi, the Vaccine Alliance. Countries participating in COVAX can access the vaccines through the COVAX Facility either as 1 of the 98 self-financing countries or, for the 92 LMICs, funded through the Gavi COVAX advance market commitment (AMC; https://www.gavi.org ).
In the current pandemic situation, the US FDA is using the EUA process to allow initial use of the vaccines from Pfizer, Moderna and Johnson & Johnson 74 . EMA is taking the approach of conditional approval 75 . The WHO emergency use assessment and listing (EUAL) procedure was developed in the wake of the Ebola virus disease outbreak in Africa to expedite the availability of vaccines. The EUAL was intended as guidance for national regulatory authorities in circumstances when the “community may be more willing to tolerate less certainty about the efficacy and safety of products, given the morbidity and/or mortality of the disease and the shortfall of treatment and/or prevention options” 76 . In early 2020, the WHO issued a revised EUL procedure to assess whether submitted data demonstrate a reasonable likelihood that a vaccine’s quality, safety and performance are acceptable and that the benefits outweigh the foreseeable risks and uncertainties in the context of a public health emergency of international concern 77 . It is intended that vaccines approved through EUAL would eventually go to full review and receive prequalification. WHO member states have the prerogative through their national regulatory authority to use the EUL procedure to authorize the use of unlicensed vaccines.
Some countries have used their national regulatory authorities to secure approval of nationally produced vaccines. The Russian government approved the Ad26 and Ad5 combination-based COVID-19 vaccine, Sputnik V, produced by the Gamaleya Research Institute, for use by individuals aged 60 years and above 78 , 79 . China’s National Medical Products Agency has given conditional approval to the whole inactivated virus BBIBP-CorV COVID-19 vaccine developed by the Beijing Institute of Biological Products, a Sinopharm subsidiary 80 . The authorization allows the general public’s use of the inoculation and comes after the company announced that its vaccine proved 79.3% effective in phase 3 trials 81 . Although the interim results are not yet published, they must have been reviewed and approved by the Chinese Center for Disease Control and Prevention and National Medical Products Agency. The United Arab Emirates was first to approve the Sinopharm vaccine for EUA in early December 2020 based on interim analysis results 82 . The Sinovac CoronaVac vaccine was recently granted conditional approval on the basis of interim efficacy results 83 . The CanSinoBIO COVID-19 vaccine achieved 65.7% efficacy in preventing symptomatic cases in clinical trials (unpublished). The vaccine also showed a 90.98% success rate in stopping severe disease in one of its interim analysis. The vaccine was granted EUA in Mexico and Pakistan 84 .
Manufacturing—how to make more, faster
Production and distribution of hundreds of millions of doses of COVID-19 vaccine within a year of identification of the pandemic pathogen is unprecedented, and while the principles are straightforward, the manufacturing equation is complex and prone to delay. The technical platform utilized to make a vaccine (mRNA, whole inactivated virus, vector, protein with or without adjuvant), the dosage (low, mid, high), the schedule of vaccination (single or two dose) and the manufacturer capability, capacity and reputation are all important considerations for regulators and the WHO. The initial phase of manufacturing scale-up will be a key regulator of vaccine access initially. This could potentially be impacted by vaccine nationalism and the announced bilateral agreements between manufacturers and high-income countries. Companies such as Sinopharm, the Serum Institute of India or Bharat have a huge capacity for production but must supply the gigantic markets of China and India. Delays in the production of several western 85 and Chinese COVID-19 vaccines 86 have already been reported.
The Developing Countries Vaccine Manufacturers Network (DCVMN) was established in 2000 with the mission to increase the availability and affordability of quality vaccines to protect against known and emerging infectious diseases 87 . About 70% of the global EPI vaccine supplies and about 75% procured by UN (United Nations) agencies are produced by DCVMN members 88 . Several technology transfers to DCVMN members have occurred over the past decades to significantly contribute to global health. Following an initial collaboration on the oral cholera vaccine between Sweden and VABIOTECH in Vietnam, the International Vaccine Institute improved the vaccine and then transferred the technology back to VABIOTECH and to several DCVMN members, including Shantha Biotechnics (Shanchol), India; EuBiologics (Euvichol), Republic of Korea; and Incepta (Cholvax), Bangladesh. Shanchol, Euvichol and Euvichol Plus are WHO prequalified and the major contributors to the Gavi-supported global stockpile 16 while Cholvax is marketed in Bangladesh.
For COVID-19 vaccines, several companies have licensed or contracted vaccine production to other manufacturers—AstraZeneca and Novavax with the Serum Institute (India) and SK Bioscience (Korea); Moderna with Lonza (Switzerland), Johnson & Johnson with Biological E (India); and Chinese Sinovac with Butantan (Brazil) and BioFarma (Indonesia). Hopefully the license and contract manufacturing arrangements will allow the production of sufficient doses of vaccines to provide equitable access to at-risk populations globally 89 .
Under the pressures of the pandemic, and with the need for accelerated development of COVID-19 vaccines, optimization of more practical aspects of vaccine implementation, supply and dosing was secondary to the need for rapid proof of concept. COVID-19 mRNA vaccines and the VSV-EBO Ebola vaccine from Merck have a similar requirement for ultracold chain storage. While that might be overcome by relatively simple technology, the scalability of these technologies for universal vaccination is unknown. Additional development is needed to establish the stability of vaccines at higher temperatures (Pfizer mRNA). There is evidence to suggest the presence of some protection against COVID-19 after the first dose; this is critical information not only for COVID-19 but also to frame thinking around other EID vaccines.
Leave no one behind, or the unequal access to vaccines and treatments
The 2030 Agenda for Sustainable Development has the vision to leave no one behind, particularly low-income countries. COVID-19 has seen exceptionalism at either extreme. On the one hand, COVAX aims to provide at least 2 billion doses of WHO-approved vaccine to participating countries by the end of 2021—roughly 20% of each country’s vaccination needs. A total of 92 LMICs will receive vaccine largely through an AMC arranged by Gavi 90 . It now appears that the USA will join COVAX, which recently announced that it had secured agreements for sufficient doses to meet the 2021 target 50 .
Critically, vaccinating people in LMICs will require additional vaccine purchases, at a cost estimated in billions of dollars. In purely economic terms, it appears that such an investment could have substantial benefit for the global economy 91 . On the other hand, COVAX is on track to achieve its goals and poised to start delivering vaccines, and yet no AMC countries had yet been vaccinated when tens of millions of people were already being vaccinated in high-income countries. Among high-income countries, billions of doses have been preordered, several times more than justified by their populations. Can COVAX achieve its target of providing 2 billion doses by 2021, or will manufacturing bottlenecks lead to delay that will allow the coronavirus to continue to circulate in poorer countries and prolong the pandemic? If unable to access COVID-19 vaccines in a timely manner, the 2030 Agenda for Sustainable Development, especially Sustainable Development Goal 3 focusing on health, will be difficult to achieve, and low-income countries will be under extraordinary pressure as the COVID-19 pandemic forces them further into poverty and deeper inequality.
UN Secretary-General António Guterres has again stressed that COVID-19 vaccines must be a global public good, available to everyone, everywhere. “Vaccinationalism is self-defeating and would delay a global recovery” 92 . Modeling studies suggest that if high-income countries take the first 2 billion doses of available COVID-19 vaccines without regard to equity, global COVID-19 deaths will double 93 . Ensuring that all countries have rapid, fair and equitable access to COVID-19 vaccines is the promise of COVAX.
Final remarks
The lessons of the COVID-19 pandemic need to be compiled and applied to the development of future vaccines against emerging infectious diseases and novel pandemic pathogens. The permanent threat of emerging pathogens calls for vigilance, surveillance and preparedness for vaccine development and deployment, all crosscutting activities to be conducted flawlessly between epidemiologists, scientists, developers, human and veterinary health authorities, regulators and funders. Global health stakeholders have learned something about developing vaccines efficiently: they still have much to learn about making and using them with due regard to equity and access.
Zhu, N. et al. A novel coronavirus from patients with pneumonia in China, 2019. N. Engl. J. Med. 382 , 727–733 (2020).
CAS PubMed PubMed Central Google Scholar
Huang, C. et al. Clinical features of patients infected with 2019 novel coronavirus in Wuhan, China. Lancet 395 , 497–506 (2020).
Wang, D. et al. Clinical characteristics of 138 hospitalized patients with 2019 novel coronavirus-infected pneumonia in Wuhan, China. JAMA 323 , 1061–1069 (2020).
Friedler, A. Sociocultural, behavioural and political factors shaping the COVID-19 pandemic: the need for a biocultural approach to understanding pandemics and (re)emerging pathogens. Glob. Public Health 16 , 17–35 (2021).
Article PubMed Google Scholar
Gully, P. R. Pandemics, regional outbreaks, and sudden-onset disasters. Healthc. Manage. Forum 33 , 164–169 (2020).
Morens, D. M. & Fauci, A. S. Emerging infectious diseases: threats to human health and global stability. PLoS Pathog. 9 , e1003467 (2013).
Article CAS PubMed PubMed Central Google Scholar
Marston, H. D., Folkers, G. K., Morens, D. M. & Fauci, A. S. Emerging viral diseases: confronting threats with new technologies. Sci. Transl. Med. 6 , 253ps210 (2014).
Article Google Scholar
Morens, D. M. & Fauci, A. S. Emerging pandemic diseases: how we got to COVID-19. Cell 182 , 1077–1092 (2020).
Morens, D. M., Daszak, P. & Taubenberger, J. K. Escaping Pandora’s box—another novel coronavirus. N. Engl. J. Med. 382 , 1293–1295 (2020).
Article CAS PubMed Google Scholar
Sacchetto, L., Drumond, B. P., Han, B. A., Nogueira, M. L. & Vasilakis, N. Re-emergence of yellow fever in the neotropics—quo vadis? Emerg. Top. Life Sci. 4 , 399–410 (2020).
PubMed Google Scholar
World Health Organization. Yellow fever. https://www.who.int/biologicals/vaccines/yellow_fever/en/ (2015).
Juan-Giner, A. et al. Immunogenicity and safety of fractional doses of yellow fever vaccines: a randomised, double-blind, non-inferiority trial. Lancet 397 , 119–127 (2021).
Prompetchara, E., Ketloy, C., Thomas, S. J. & Ruxrungtham, K. Dengue vaccine: global development update. Asian Pac. J. Allergy Immunol. 38 , 178–185 (2020).
PubMed CAS Google Scholar
Thomas, S. J. & Yoon, I. K. A review of Dengvaxia ® : development to deployment. Hum. Vaccin. Immunother. 15 , 2295–2314 (2019).
Article PubMed PubMed Central Google Scholar
Hu, D. et al. Origins of the current seventh cholera pandemic. Proc. Natl Acad. Sci. USA 113 , E7730–E7739 (2016).
Shaikh, H., Lynch, J., Kim, J. & Excler, J. L. Current and future cholera vaccines. Vaccine 38 , A118–A126 (2020).
Klemm, E. J. et al. Emergence of an extensively drug-resistant Salmonella enterica serovar Typhi clone harboring a promiscuous plasmid encoding resistance to fluoroquinolones and third-generation cephalosporins. mBio 9 , e00105–18 (2018).
Yousafzai, M. T. et al. Ceftriaxone-resistant Salmonella Typhi outbreak in Hyderabad City of Sindh, Pakistan: high time for the introduction of typhoid conjugate vaccine. Clin. Infect. Dis. 68 , S16–S21 (2019).
Qamar, F. N. et al. Antimicrobial resistance in typhoidal salmonella: surveillance for enteric fever in Asia project, 2016–2019. Clin. Infect. Dis. 71 , S276–S284 (2020).
Marchello, C. S., Carr, S. D. & Crump, J. A. A systematic review on antimicrobial resistance among Salmonella Typhi worldwide. Am. J. Trop. Med. Hyg. 103 , 2518–2527 (2020).
Andrews, J. R. et al. Typhoid conjugate vaccines: a new tool in the fight against antimicrobial resistance. Lancet Infect. Dis. 19 , e26–e30 (2019).
D’Souza, M. P. & Frahm, N. Adenovirus 5 serotype vector-specific immunity and HIV-1 infection: a tale of T cells and antibodies. AIDS 24 , 803–809 (2010).
Lin, J. T. et al. Safety and immunogenicity from a phase I trial of inactivated severe acute respiratory syndrome coronavirus vaccine. Antivir. Ther. 12 , 1107–1113 (2007).
Martin, J. E. et al. A SARS DNA vaccine induces neutralizing antibody and cellular immune responses in healthy adults in a Phase I clinical trial. Vaccine 26 , 6338–6343 (2008).
Jacob, S. T. et al. Ebola virus disease. Nat. Rev. Dis. Primers 6 , 13 (2020).
Wolf, J. et al. Applying lessons from the Ebola vaccine experience for SARS-CoV-2 and other epidemic pathogens. NPJ Vaccines 5 , 51 (2020).
Ball, P. The lightning-fast quest for COVID vaccines—and what it means for other diseases. Nature 589 , 16–18 (2021).
Feldmann, H., Feldmann, F. & Marzi, A. Ebola: lessons on vaccine development. Annu. Rev. Microbiol. 72 , 423–446 (2018).
Gouglas, D., Christodoulou, M., Plotkin, S. A. & Hatchett, R. CEPI: driving progress toward epidemic preparedness and response. Epidemiol. Rev. 41 , 28–33 (2019).
Rottingen, J. A. et al. New vaccines against epidemic infectious diseases. N. Engl. J. Med. 376 , 610–613 (2017).
Sandbrink, J. B. & Shattock, R. J. RNA vaccines: a suitable platform for tackling emerging pandemics? Front. Immunol. 11 , 608460 (2020).
Jackson, N. A. C., Kester, K. E., Casimiro, D., Gurunathan, S. & DeRosa, F. The promise of mRNA vaccines: a biotech and industrial perspective. NPJ Vaccines 5 , 11 (2020).
US Department of Health and Human Health Services. Explaining Operation Warp Speed. https://health.mo.gov/living/healthcondiseases/communicable/novel-coronavirus-lpha/pdf/fact-sheet-operation-warp-speed.pdf 2020).
van Riel, D. & de Wit, E. Next-generation vaccine platforms for COVID-19. Nat. Mater. 19 , 810–812 (2020).
Article PubMed CAS Google Scholar
Polack, F. P. et al. Safety and efficacy of the BNT162b2 mRNA Covid-19 vaccine. N. Engl. J. Med. 383 , 2603–2615 (2020).
Baden, L. R. et al. Efficacy and safety of the mRNA-1273 SARS-CoV-2 vaccine. N. Engl. J. Med. 384 , 403–416 (2021).
US Food and Drug Administration. FDA takes key action in fight against COVID-19 by issuing emergency use authorization for first COVID-19 vaccine. https://www.fda.gov/news-events/press-announcements/fda-takes-key-action-fight-against-covid-19-issuing-emergency-use-authorization-first-covid-19 (2020).
US Food and Drug Administration. FDA takes additional action in fight against COVID-19 by issuing emergency use authorization for second COVID-19 vaccine. https://www.fda.gov/news-events/press-announcements/fda-takes-additional-action-fight-against-covid-19-issuing-emergency-use-authorization-second-covid (2020).
European Medicines Agency. EMA recommends first COVID-19 vaccine for authorisation in the EU. https://www.ema.europa.eu/en/news/ema-recommends-first-covid-19-vaccine-authorisation-eu (2020).
European Medicines Agency. EMA recommends COVID-19 Vaccine Moderna for authorisation in the EU. https://www.ema.europa.eu/en/news/ema-recommends-covid-19-vaccine-moderna-authorisation-eu (2021).
Liu, M. A. A Comparison of plasmid DNA and mRNA as vaccine technologies. Vaccines 7 , 37 (2019).
Article CAS PubMed Central Google Scholar
Pollard, A. J. et al. Safety and immunogenicity of a two-dose heterologous Ad26.ZEBOV and MVA-BN-Filo Ebola vaccine regimen in adults in Europe (EBOVAC2): a randomised, observer-blind, participant-blind, placebo-controlled, phase 2 trial. Lancet Infect. Dis . https://doi.org/10.1016/S1473-3099(20)30476-X (2020).
Adalja, A. A., Watson, M., Cicero, A. & Inglesby, T. Vaccine platform technologies: a potent tool for emerging infectious disease vaccine development. Health Secur. 18 , 59–60 (2020).
Excler, J. L. & Kim, J. H. Novel prime-boost vaccine strategies against HIV-1. Expert Rev. Vaccines 18 , 765–779 (2019).
European Pharmaceutical Review. AstraZeneca to test combination of AZD1222 and Sputnik V vaccines. https://www.europeanpharmaceuticalreview.com/news/136683/astrazeneca-to-test-combination-of-azd1222-and-sputnik-v-vaccines/ (2020).
World Health Organization. Draft landscape and tracker of COVID-19 candidate vaccines. https://www.who.int/publications/m/item/draft-landscape-of-covid-19-candidate-vaccines (2021).
Fritzell, B. Bridging studies. Dev. Biol. Stand. 95 , 181–188 (1998).
CAS PubMed Google Scholar
WHO Ad Hoc Expert Group on the Next Steps for Covid-19 Vaccine Evaluation. Placebo-controlled trials of Covid-19 vaccines—why we still need them. N. Engl. J. Med . 384 , e2 (2021).
Clemens, J., Brenner, R., Rao, M., Tafari, N. & Lowe, C. Evaluating new vaccines for developing countries. Efficacy or effectiveness? JAMA 275 , 390–397 (1996).
Kim, J. H., Marks, F. & Clemens, J. D. Looking beyond COVID-19 vaccine phase 3 trials. Nat. Med. 27 , 205–211 (2021).
Follmann, D. et al. Assessing durability of vaccine effect following blinded crossover in COVID-19 vaccine efficacy trials. Preprint at https://www.medrxiv.org/content/10.1101/2020.12.14.20248137v1 (2020).
World Health Organization. Global Advisory Committee on Vaccine Safety, 27–28 May 2020. https://www.who.int/vaccine_safety/committee/reports/May_2020/en/ (2020).
World Health Organization. COVID-19 vaccines: safety surveillance manual. https://apps.who.int/iris/handle/10665/338400 (2020).
European Medicines Agency. Pharmacovigilance plan of the EU Regulatory Network for COVID-19 Vaccines. https://www.ema.europa.eu/en/documents/other/pharmacovigilance-plan-eu-regulatory-network-covid-19-vaccines_en.pdf (2020).
Lee, G. M., Romero, J. R. & Bell, B. P. Postapproval vaccine safety surveillance for COVID-19 vaccines in the US. JAMA 324 , 1937–1938 (2020).
Kieny, M. P. & Rago, L. Regulatory policy for research and development of vaccines for public health emergencies. Expert Rev. Vaccines 15 , 1075–1077 (2016).
Lambert, P. H. et al. Consensus summary report for CEPI/BC March 12–13, 2020 meeting: assessment of risk of disease enhancement with COVID-19 vaccines. Vaccine 38 , 4783–4791 (2020).
Zellweger, R. M., Wartel, T. A., Marks, F., Song, M. & Kim, J. H. Vaccination against SARS-CoV-2 and disease enhancement - knowns and unknowns. Expert Rev. Vaccines 19 , 691–698 (2020).
Creanga, A. A. et al. Severity of 2009 pandemic influenza A (H1N1) virus infection in pregnant women. Obstet. Gynecol. 115 , 717–726 (2010).
Menendez, C., Lucas, A., Munguambe, K. & Langer, A. Ebola crisis: the unequal impact on women and children’s health. Lancet Glob. Health 3 , e130 (2015).
The PREVENT Working Group. Pregnant women and vaccines against emerging epidemic threats: ethics guidance on preparedness, research and response. http://vax.pregnancyethics.org/prevent-guidance (2018).
Krubiner, C. B. et al. Pregnant women and vaccines against emerging epidemic threats: ethics guidance for preparedness, research, and response. Vaccine 39 , 85–120 (2021).
Edridge, A. W. D. et al. Seasonal coronavirus protective immunity is short-lasting. Nat. Med. 26 , 1691–1693 (2020).
Plante, J. A. et al. Spike mutation D614G alters SARS-CoV-2 fitness. Nature https://doi.org/10.1038/s41586-020-2895-3 (2020).
Korber, B. et al. Tracking changes in SARS-CoV-2 spike: evidence that D614G increases infectivity of the COVID-19 virus. Cell 182 , 812–827 (2020).
Hou, Y. J. et al. SARS-CoV-2 D614G variant exhibits efficient replication ex vivo and transmission in vivo. Science 370 , 1464–1468 (2020).
Volz, E. et al. Evaluating the effects of SARS-CoV-2 spike mutation D614G on transmissibility and pathogenicity. Cell 184 , 64–75.e11 (2021).
Faria, N. R. Genomic characterisation of an emergent SARS-CoV-2 lineage in Manaus: preliminary findings. Virological https://virological.org/t/586 (2020).
Wibmer, C. K. et al. SARS-CoV-2 501Y.V2 escapes neutralization by South African COVID-19 donor plasma. Preprint at bioRxiv https://doi.org/10.1101/2021.01.18.427166 (2021).
BBC. Covid: South Africa halts AstraZeneca vaccine rollout over new variant. https://www.bbc.com/news/world-africa-55975052 (2021).
Mahase, E. Covid-19: Novavax vaccine efficacy is 86% against UK variant and 60% against South African variant. BMJ 372 , n296 (2021).
Johnson & Johnson. Johnson & Johnson announces single-shot Janssen COVID-19 vaccine candidate met primary endpoints in interim analysis of its phase 3 ENSEMBLE trial. https://www.jnj.com/johnson-johnson-announces-single-shot-janssen-covid-19-vaccine-candidate-met-primary-endpoints-in-interim-analysis-of-its-phase-3-ensemble-trial (2021).
World Health Organization. Global Influenza Surveillance and Response System (GISRS). https://www.who.int/influenza/gisrs_laboratory/en/
US Food and Drug Administration. Emergency use authorization. https://www.fda.gov/emergency-preparedness-and-response/mcm-legal-regulatory-and-policy-framework/emergency-use-authorization (2021).
European Medicines Agency. Conditional marketing authorization. https://www.ema.europa.eu/en/human-regulatory/marketing-authorisation/conditional-marketing-authorisation (2020).
Smith, M. J., Ujewe, S., Katz, R. & Upshur, R. E. G. Emergency use authorisation for COVID-19 vaccines: lessons from Ebola. Lancet 396 , 1707–1709 (2020).
World Health Organization. Emergency use listing procedure. https://www.who.int/publications/m/item/emergency-use-listing-procedure (2020).
Pharmaceutical Technology. Russia approves Sputnik V Covid-19 vaccine for senior citizens. https://www.pharmaceutical-technology.com/news/russia-sputnik-senior-citizens/ (2021).
Logunov, D. Y. et al. Safety and efficacy of an rAd26 and rAd5 vector-based heterologous prime-boost COVID-19 vaccine: an interim analysis of a randomised controlled phase 3 trial in Russia. Lancet https://doi.org/10.1016/S0140-6736(21)00234-8 (2021).
Xinhuanet. China approves first self-developed COVID-19 vaccine. http://www.xinhuanet.com/english/2020-12/31/c_139632402.htm (2020).
Sinopharm. China grants conditional market approval for Sinopharm CNBG’s COVID-19 vaccine. http://www.sinopharm.com/en/s/1395-4173-38862.html (2021).
CNBC. Dubai is offering the Pfizer vaccine to residents for free in addition to China’s Sinopharm shot. https://www.cnbc.com/2020/12/23/dubai-offering-pfizer-sinopharm-covid-vaccines-to-residents-for-free.html (2020).
Reuters. China approves Sinovac Biotech COVID-19 vaccine for general public use. https://www.reuters.com/article/us-health-coronavirus-vaccine-sinovac-idUSKBN2A60AY (2021).
Reuters. Pakistan approves Chinese CanSinoBIO COVID vaccine for emergency use. https://www.reuters.com/article/us-health-coronavirus-pakistan-vaccine/pakistan-approves-chinese-cansinobio-covid-vaccine-for-emergency-use-idUSKBN2AC1FG (2021).
Euronews. Coronavirus: 15m people in the UK have now had their first COVID jab—what about wider Europe? https://www.euronews.com/2021/02/14/coronavirus-15m-people-in-the-uk-have-now-had-their-first-covid-jab-what-about-wider-europ (2021).
Deng, C. & Malsin, J. China’s Covid-19 vaccine makers struggle to meet demand. The Wall Street Journal https://www.wsj.com/articles/chinas-covid-19-vaccine-makers-struggle-to-meet-demand-11612958560 (10 February 2021).
Pagliusi, S. et al. Developing countries vaccine manufacturers network: doing good by making high-quality vaccines affordable for all. Vaccine 31 , B176–B183 (2013).
Jadhav, S., Gautam, M. & Gairola, S. Role of vaccine manufacturers in developing countries towards global healthcare by providing quality vaccines at affordable prices. Clin. Microbiol. Infect. 20 , 37–44 (2014).
Kim, J. H. SARS-CoV-2 vaccine development, access, and equity. J. Exp. Med. 217 , e20201288 (2020).
Nhamo, G., Chikodzi, D., Kunene, H. P. & Mashula, N. COVID-19 vaccines and treatments nationalism: challenges for low-income countries and the attainment of the SDGs. Glob. Public Health 16 , 319–339 (2020).
World Bank. Global economy to expand by 4% in 2021; vaccine deployment and investment key to sustaining the recovery. https://www.worldbank.org/en/news/press-release/2021/01/05/global-economy-to-expand-by-4-percent-in-2021-vaccine-deployment-and-investment-key-to-sustaining-the-recovery (2021).
UN News. WHO chief warns against ‘catastrophic moral failure’ in COVID-19 vaccine access. https://news.un.org/en/story/2021/01/1082362 (18 January 2021).
Chinazzi, M. et al. The effect of travel restrictions on the spread of the 2019 novel coronavirus (COVID-19) outbreak. Science 368 , 395–400 (2020).
Download references
Author information
Authors and affiliations.
International Vaccine Institute, Seoul, Republic of Korea
Jean-Louis Excler & Jerome H. Kim
Coalition for Epidemic Preparedness Innovations (CEPI), London, UK
Melanie Saville
Gavi, the Vaccine Alliance, Geneva, Switzerland
Seth Berkley
You can also search for this author in PubMed Google Scholar
Contributions
J.-L.E., M.S., S.B. and J.H.K. equally contributed to the synopsis of the manuscript. J.-L.E. and J.H.K. wrote the text and tables of the manuscript. J.H.K. provided the figure. M.S. and S.B. edited the manuscript.
Corresponding authors
Correspondence to Jean-Louis Excler or Jerome H. Kim .
Ethics declarations
Competing interests.
J.-L.E. is a consultant for vaccine safety for the Brighton Collaboration, Johnson & Johnson and the US Military HIV Research Program. J.H.K. is a consultant to SK Bioscience. M.S. has a financial interest in Sanofi (shares). S.B. does not have any financial or nonfinancial conflicts of interest.
Additional information
Publisher’s note Springer Nature remains neutral with regard to jurisdictional claims in published maps and institutional affiliations.
Peer review information Nature Medicine thanks Robert Carnahan and David Morens for their contribution to the peer review of this work. Joao Monteiro was the primary editor on this article and managed its editorial process and peer review in collaboration with the rest of the editorial team.
Rights and permissions
Reprints and permissions
About this article
Cite this article.
Excler, JL., Saville, M., Berkley, S. et al. Vaccine development for emerging infectious diseases. Nat Med 27 , 591–600 (2021). https://doi.org/10.1038/s41591-021-01301-0
Download citation
Received : 27 January 2021
Accepted : 01 March 2021
Published : 12 April 2021
Issue Date : April 2021
DOI : https://doi.org/10.1038/s41591-021-01301-0
Share this article
Anyone you share the following link with will be able to read this content:
Sorry, a shareable link is not currently available for this article.
Provided by the Springer Nature SharedIt content-sharing initiative
This article is cited by
Parental hesitancy toward children vaccination: a multi-country psychometric and predictive study.
- Hamid Sharif-Nia
- Fatemeh Khoshnavay Fomani
BMC Public Health (2024)
Current landscape of mRNA technologies and delivery systems for new modality therapeutics
- Ruei-Min Lu
- Hsiang-En Hsu
- Han-Chung Wu
Journal of Biomedical Science (2024)
Empowering standardization of cancer vaccines through ontology: enhanced modeling and data analysis
- Xingxian Li
Journal of Biomedical Semantics (2024)
Assessment of immunopathological responses of a novel non-chemical biocide in C57BL/6 for safe disinfection usage
- Keun Bon Ku
- Jihwan Chae
- Heung Kyu Lee
Laboratory Animal Research (2024)
Nanotechnology’s frontier in combatting infectious and inflammatory diseases: prevention and treatment
- Yujing Huang
- Xiaohan Guo
Signal Transduction and Targeted Therapy (2024)
Quick links
- Explore articles by subject
- Guide to authors
- Editorial policies
Sign up for the Nature Briefing: Microbiology newsletter — what matters in microbiology research, free to your inbox weekly.

