JMP | Statistical Discovery.™ From SAS.

Statistics Knowledge Portal
A free online introduction to statistics
Chi-Square Test of Independence
What is the chi-square test of independence.
The Chi-square test of independence is a statistical hypothesis test used to determine whether two categorical or nominal variables are likely to be related or not.
When can I use the test?
You can use the test when you have counts of values for two categorical variables.
Can I use the test if I have frequency counts in a table?
Yes. If you have only a table of values that shows frequency counts, you can use the test.
Using the Chi-square test of independence
See how to perform a chi-square test of independence using statistical software.
- Download JMP to follow along using the sample data included with the software.
- To see more JMP tutorials, visit the JMP Learning Library .
The Chi-square test of independence checks whether two variables are likely to be related or not. We have counts for two categorical or nominal variables. We also have an idea that the two variables are not related. The test gives us a way to decide if our idea is plausible or not.
The sections below discuss what we need for the test, how to do the test, understanding results, statistical details and understanding p-values.
What do we need?
For the Chi-square test of independence, we need two variables. Our idea is that the variables are not related. Here are a couple of examples:
- We have a list of movie genres; this is our first variable. Our second variable is whether or not the patrons of those genres bought snacks at the theater. Our idea (or, in statistical terms, our null hypothesis) is that the type of movie and whether or not people bought snacks are unrelated. The owner of the movie theater wants to estimate how many snacks to buy. If movie type and snack purchases are unrelated, estimating will be simpler than if the movie types impact snack sales.
- A veterinary clinic has a list of dog breeds they see as patients. The second variable is whether owners feed dry food, canned food or a mixture. Our idea is that the dog breed and types of food are unrelated. If this is true, then the clinic can order food based only on the total number of dogs, without consideration for the breeds.
For a valid test, we need:
- Data values that are a simple random sample from the population of interest.
- Two categorical or nominal variables. Don't use the independence test with continous variables that define the category combinations. However, the counts for the combinations of the two categorical variables will be continuous.
- For each combination of the levels of the two variables, we need at least five expected values. When we have fewer than five for any one combination, the test results are not reliable.
Chi-square test of independence example
Let’s take a closer look at the movie snacks example. Suppose we collect data for 600 people at our theater. For each person, we know the type of movie they saw and whether or not they bought snacks.
Let’s start by answering: Is the Chi-square test of independence an appropriate method to evaluate the relationship between movie type and snack purchases?
- We have a simple random sample of 600 people who saw a movie at our theater. We meet this requirement.
- Our variables are the movie type and whether or not snacks were purchased. Both variables are categorical. We meet this requirement.
- The last requirement is for more than five expected values for each combination of the two variables. To confirm this, we need to know the total counts for each type of movie and the total counts for whether snacks were bought or not. For now, we assume we meet this requirement and will check it later.
It appears we have indeed selected a valid method. (We still need to check that more than five values are expected for each combination.)
Here is our data summarized in a contingency table:
Table 1: Contingency table for movie snacks data
Before we go any further, let’s check the assumption of five expected values in each category. The data has more than five counts in each combination of Movie Type and Snacks. But what are the expected counts if movie type and snack purchases are independent?
Finding expected counts
To find expected counts for each Movie-Snack combination, we first need the row and column totals, which are shown below:
Table 2: Contingency table for movie snacks data with row and column totals
The expected counts for each Movie-Snack combination are based on the row and column totals. We multiply the row total by the column total and then divide by the grand total. This gives us the expected count for each cell in the table. For example, for the Action-Snacks cell, we have:
$ \frac{125\times310}{600} = \frac{38,750}{600} = 65 $
We rounded the answer to the nearest whole number. If there is not a relationship between movie type and snack purchasing we would expect 65 people to have watched an action film with snacks.
Here are the actual and expected counts for each Movie-Snack combination. In each cell of Table 3 below, the expected count appears in bold beneath the actual count. The expected counts are rounded to the nearest whole number.
Table 3: Contingency table for movie snacks data showing actual count vs. expected count
When using software, these calculated values will be labeled as “expected values,” “expected cell counts” or some similar term.
All of the expected counts for our data are larger than five, so we meet the requirement for applying the independence test.
Before calculating the test statistic, let’s look at the contingency table again. The expected counts use the row and column totals. If we look at each of the cells, we can see that some expected counts are close to the actual counts but most are not. If there is no relationship between the movie type and snack purchases, the actual and expected counts will be similar. If there is a relationship, the actual and expected counts will be different.
A common mistake with expected counts is to simply divide the grand total by the number of cells. For our movie data, this is 600 / 8 = 75. This is not correct. We know the row totals and column totals. These are fixed and cannot change for our data. The expected values are based on the row and column totals, not just on the grand total.
Performing the test
The basic idea in calculating the test statistic is to compare actual and expected values, given the row and column totals that we have in the data. First, we calculate the difference from actual and expected for each Movie-Snacks combination. Next, we square that difference. Squaring gives the same importance to combinations with fewer actual values than expected and combinations with more actual values than expected. Next, we divide by the expected value for the combination. We add up these values for each Movie-Snacks combination. This gives us our test statistic.
This is much easier to follow using the data from our example. Table 4 below shows the calculations for each Movie-Snacks combination carried out to two decimal places.
Table 4: Preparing to calculate our test statistic
Lastly, to get our test statistic, we add the numbers in the final row for each cell:
$ 3.29 + 3.52 + 5.81 + 6.21 + 12.65 + 13.52 + 9.68 + 10.35 = 65.03 $
To make our decision, we compare the test statistic to a value from the Chi-square distribution . This activity involves five steps:
- We decide on the risk we are willing to take of concluding that the two variables are not independent when in fact they are. For the movie data, we had decided prior to our data collection that we are willing to take a 5% risk of saying that the two variables – Movie Type and Snack Purchase – are not independent when they really are independent. In statistics-speak, we set the significance level, α, to 0.05.
- We calculate a test statistic. As shown above, our test statistic is 65.03.
- We find the critical value from the Chi-square distribution based on our degrees of freedom and our significance level. This is the value we expect if the two variables are independent.
- The degrees of freedom depend on how many rows and how many columns we have. The degrees of freedom (df) are calculated as: $ \text{df} = (r-1)\times(c-1) $ In the formula, r is the number of rows, and c is the number of columns in our contingency table. From our example, with Movie Type as the rows and Snack Purchase as the columns, we have: $ \text{df} = (4-1)\times(2-1) = 3\times1 = 3 $ The Chi-square value with α = 0.05 and three degrees of freedom is 7.815.
- We compare the value of our test statistic (65.03) to the Chi-square value. Since 65.03 > 7.815, we reject the idea that movie type and snack purchases are independent.
We conclude that there is some relationship between movie type and snack purchases. The owner of the movie theater cannot estimate how many snacks to buy regardless of the type of movies being shown. Instead, the owner must think about the type of movies being shown when estimating snack purchases.
It's important to note that we cannot conclude that the type of movie causes a snack purchase. The independence test tells us only whether there is a relationship or not; it does not tell us that one variable causes the other.
Understanding results
Let’s use graphs to understand the test and the results.
The side-by-side chart below shows the actual counts in blue, and the expected counts in orange. The counts appear at the top of the bars. The yellow box shows the movie type and snack purchase totals. These totals are needed to find the expected counts.
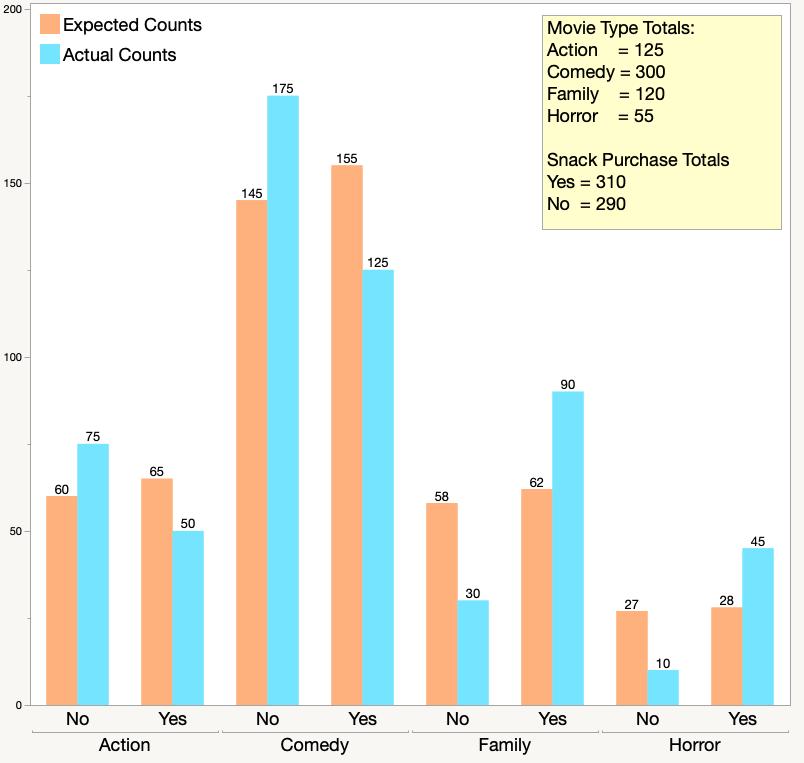
Compare the expected and actual counts for the Horror movies. You can see that more people than expected bought snacks and fewer people than expected chose not to buy snacks.
If you look across all four of the movie types and whether or not people bought snacks, you can see that there is a fairly large difference between actual and expected counts for most combinations. The independence test checks to see if the actual data is “close enough” to the expected counts that would occur if the two variables are independent. Even without a statistical test, most people would say that the two variables are not independent. The statistical test provides a common way to make the decision, so that everyone makes the same decision on the data.
The chart below shows another possible set of data. This set has the exact same row and column totals for movie type and snack purchase, but the yes/no splits in the snack purchase data are different.
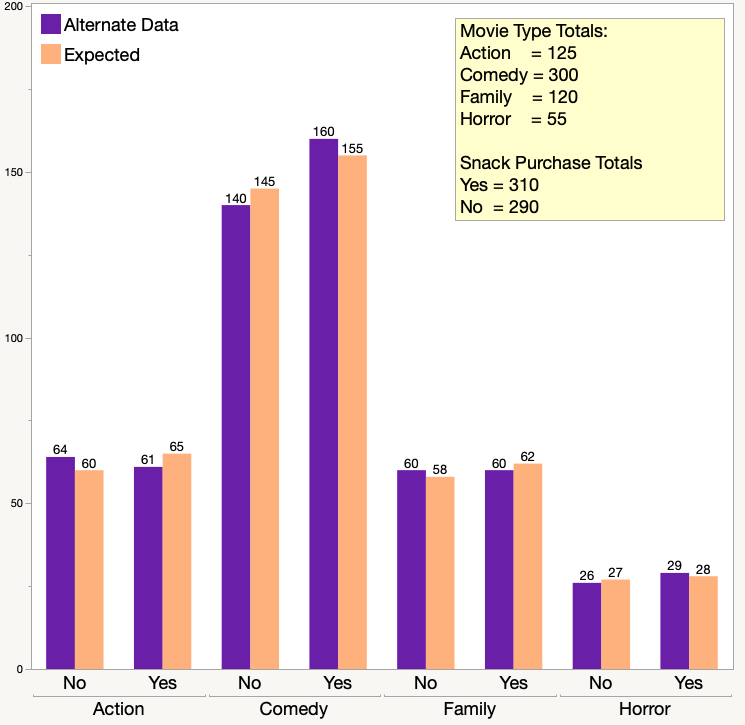
The purple bars show the actual counts in this data. The orange bars show the expected counts, which are the same as in our original data set. The expected counts are the same because the row totals and column totals are the same. Looking at the graph above, most people would think that the type of movie and snack purchases are independent. If you perform the Chi-square test of independence using this new data, the test statistic is 0.903. The Chi-square value is still 7.815 because the degrees of freedom are still three. You would fail to reject the idea of independence because 0.903 < 7.815. The owner of the movie theater can estimate how many snacks to buy regardless of the type of movies being shown.
Statistical details
Let’s look at the movie-snack data and the Chi-square test of independence using statistical terms.
Our null hypothesis is that the type of movie and snack purchases are independent. The null hypothesis is written as:
$ H_0: \text{Movie Type and Snack purchases are independent} $
The alternative hypothesis is the opposite.
$ H_a: \text{Movie Type and Snack purchases are not independent} $
Before we calculate the test statistic, we find the expected counts. This is written as:
$ Σ_{ij} = \frac{R_i\times{C_j}}{N} $
The formula is for an i x j contingency table. That is a table with i rows and j columns. For example, E 11 is the expected count for the cell in the first row and first column. The formula shows R i as the row total for the i th row, and C j as the column total for the j th row. The overall sample size is N .
We calculate the test statistic using the formula below:
$ Σ^n_{i,j=1} = \frac{(O_{ij}-E_{ij})^2}{E_{ij}} $
In the formula above, we have n combinations of rows and columns. The Σ symbol means to add up the calculations for each combination. (We performed these same steps in the Movie-Snack example, beginning in Table 4.) The formula shows O ij as the Observed count for the ij -th combination and E i j as the Expected count for the combination. For the Movie-Snack example, we had four rows and two columns, so we had eight combinations.
We then compare the test statistic to the critical Chi-square value corresponding to our chosen alpha value and the degrees of freedom for our data. Using the Movie-Snack data as an example, we had set α = 0.05 and had three degrees of freedom. For the Movie-Snack data, the Chi-square value is written as:
$ χ_{0.05,3}^2 $
There are two possible results from our comparison:
- The test statistic is lower than the Chi-square value. You fail to reject the hypothesis of independence. In the movie-snack example, the theater owner can go ahead with the assumption that the type of movie a person sees has no relationship with whether or not they buy snacks.
- The test statistic is higher than the Chi-square value. You reject the hypothesis of independence. In the movie-snack example, the theater owner cannot assume that there is no relationship between the type of movie a person sees and whether or not they buy snacks.
Understanding p-values
Let’s use a graph of the Chi-square distribution to better understand the p-values. You are checking to see if your test statistic is a more extreme value in the distribution than the critical value. The graph below shows a Chi-square distribution with three degrees of freedom. It shows how the value of 7.815 “cuts off” 95% of the data. Only 5% of the data from a Chi-square distribution with three degrees of freedom is greater than 7.815.
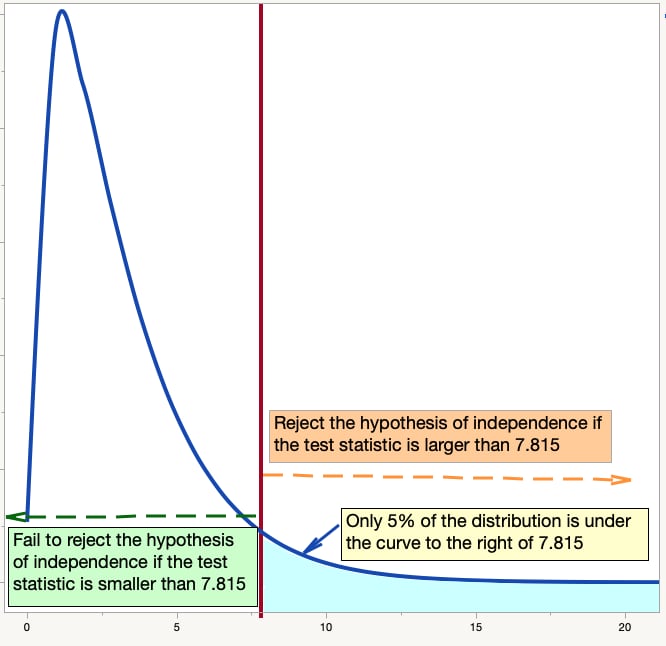
The next distribution graph shows our results. You can see how far out “in the tail” our test statistic is. In fact, with this scale, it looks like the distribution curve is at zero at the point at which it intersects with our test statistic. It isn’t, but it is very, very close to zero. We conclude that it is very unlikely for this situation to happen by chance. The results that we collected from our movie goers would be extremely unlikely if there were truly no relationship between types of movies and snack purchases.
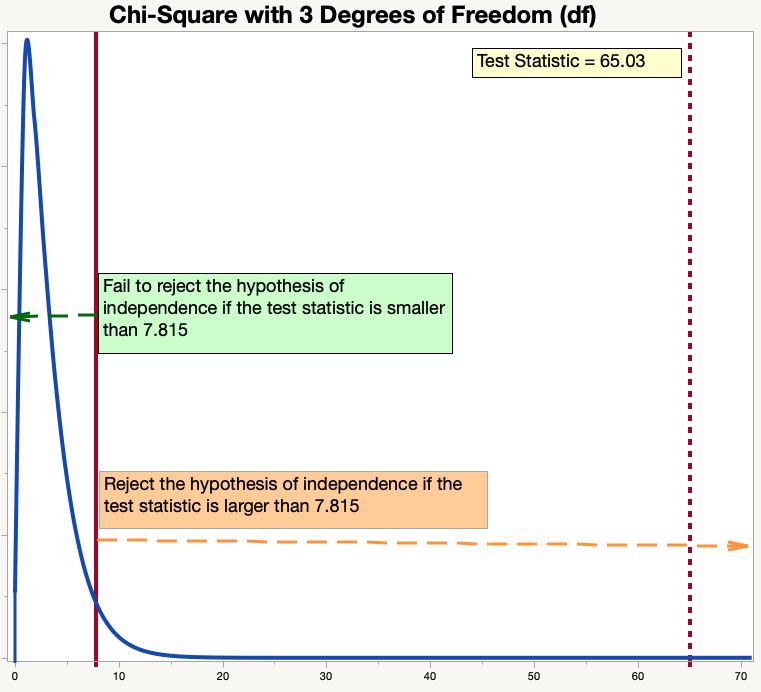
Statistical software shows the p-value for a test. This is the likelihood of another sample of the same size resulting in a test statistic more extreme than the test statistic from our current sample, assuming that the null hypothesis is true. It’s difficult to calculate this by hand. For the distributions shown above, if the test statistic is exactly 7.815, then the p - value will be p=0.05. With the test statistic of 65.03, the p - value is very, very small. In this example, most statistical software will report the p - value as “p < 0.0001.” This means that the likelihood of finding a more extreme value for the test statistic using another random sample (and assuming that the null hypothesis is correct) is less than one chance in 10,000.
Hypothesis Testing - Chi Squared Test
Lisa Sullivan, PhD
Professor of Biostatistics
Boston University School of Public Health

Introduction
This module will continue the discussion of hypothesis testing, where a specific statement or hypothesis is generated about a population parameter, and sample statistics are used to assess the likelihood that the hypothesis is true. The hypothesis is based on available information and the investigator's belief about the population parameters. The specific tests considered here are called chi-square tests and are appropriate when the outcome is discrete (dichotomous, ordinal or categorical). For example, in some clinical trials the outcome is a classification such as hypertensive, pre-hypertensive or normotensive. We could use the same classification in an observational study such as the Framingham Heart Study to compare men and women in terms of their blood pressure status - again using the classification of hypertensive, pre-hypertensive or normotensive status.
The technique to analyze a discrete outcome uses what is called a chi-square test. Specifically, the test statistic follows a chi-square probability distribution. We will consider chi-square tests here with one, two and more than two independent comparison groups.
Learning Objectives
After completing this module, the student will be able to:
- Perform chi-square tests by hand
- Appropriately interpret results of chi-square tests
- Identify the appropriate hypothesis testing procedure based on type of outcome variable and number of samples
Tests with One Sample, Discrete Outcome
Here we consider hypothesis testing with a discrete outcome variable in a single population. Discrete variables are variables that take on more than two distinct responses or categories and the responses can be ordered or unordered (i.e., the outcome can be ordinal or categorical). The procedure we describe here can be used for dichotomous (exactly 2 response options), ordinal or categorical discrete outcomes and the objective is to compare the distribution of responses, or the proportions of participants in each response category, to a known distribution. The known distribution is derived from another study or report and it is again important in setting up the hypotheses that the comparator distribution specified in the null hypothesis is a fair comparison. The comparator is sometimes called an external or a historical control.
In one sample tests for a discrete outcome, we set up our hypotheses against an appropriate comparator. We select a sample and compute descriptive statistics on the sample data. Specifically, we compute the sample size (n) and the proportions of participants in each response
Test Statistic for Testing H 0 : p 1 = p 10 , p 2 = p 20 , ..., p k = p k0
We find the critical value in a table of probabilities for the chi-square distribution with degrees of freedom (df) = k-1. In the test statistic, O = observed frequency and E=expected frequency in each of the response categories. The observed frequencies are those observed in the sample and the expected frequencies are computed as described below. χ 2 (chi-square) is another probability distribution and ranges from 0 to ∞. The test above statistic formula above is appropriate for large samples, defined as expected frequencies of at least 5 in each of the response categories.
When we conduct a χ 2 test, we compare the observed frequencies in each response category to the frequencies we would expect if the null hypothesis were true. These expected frequencies are determined by allocating the sample to the response categories according to the distribution specified in H 0 . This is done by multiplying the observed sample size (n) by the proportions specified in the null hypothesis (p 10 , p 20 , ..., p k0 ). To ensure that the sample size is appropriate for the use of the test statistic above, we need to ensure that the following: min(np 10 , n p 20 , ..., n p k0 ) > 5.
The test of hypothesis with a discrete outcome measured in a single sample, where the goal is to assess whether the distribution of responses follows a known distribution, is called the χ 2 goodness-of-fit test. As the name indicates, the idea is to assess whether the pattern or distribution of responses in the sample "fits" a specified population (external or historical) distribution. In the next example we illustrate the test. As we work through the example, we provide additional details related to the use of this new test statistic.
A University conducted a survey of its recent graduates to collect demographic and health information for future planning purposes as well as to assess students' satisfaction with their undergraduate experiences. The survey revealed that a substantial proportion of students were not engaging in regular exercise, many felt their nutrition was poor and a substantial number were smoking. In response to a question on regular exercise, 60% of all graduates reported getting no regular exercise, 25% reported exercising sporadically and 15% reported exercising regularly as undergraduates. The next year the University launched a health promotion campaign on campus in an attempt to increase health behaviors among undergraduates. The program included modules on exercise, nutrition and smoking cessation. To evaluate the impact of the program, the University again surveyed graduates and asked the same questions. The survey was completed by 470 graduates and the following data were collected on the exercise question:
Based on the data, is there evidence of a shift in the distribution of responses to the exercise question following the implementation of the health promotion campaign on campus? Run the test at a 5% level of significance.
In this example, we have one sample and a discrete (ordinal) outcome variable (with three response options). We specifically want to compare the distribution of responses in the sample to the distribution reported the previous year (i.e., 60%, 25%, 15% reporting no, sporadic and regular exercise, respectively). We now run the test using the five-step approach.
- Step 1. Set up hypotheses and determine level of significance.
The null hypothesis again represents the "no change" or "no difference" situation. If the health promotion campaign has no impact then we expect the distribution of responses to the exercise question to be the same as that measured prior to the implementation of the program.
H 0 : p 1 =0.60, p 2 =0.25, p 3 =0.15, or equivalently H 0 : Distribution of responses is 0.60, 0.25, 0.15
H 1 : H 0 is false. α =0.05
Notice that the research hypothesis is written in words rather than in symbols. The research hypothesis as stated captures any difference in the distribution of responses from that specified in the null hypothesis. We do not specify a specific alternative distribution, instead we are testing whether the sample data "fit" the distribution in H 0 or not. With the χ 2 goodness-of-fit test there is no upper or lower tailed version of the test.
- Step 2. Select the appropriate test statistic.
The test statistic is:
We must first assess whether the sample size is adequate. Specifically, we need to check min(np 0 , np 1, ..., n p k ) > 5. The sample size here is n=470 and the proportions specified in the null hypothesis are 0.60, 0.25 and 0.15. Thus, min( 470(0.65), 470(0.25), 470(0.15))=min(282, 117.5, 70.5)=70.5. The sample size is more than adequate so the formula can be used.
- Step 3. Set up decision rule.
The decision rule for the χ 2 test depends on the level of significance and the degrees of freedom, defined as degrees of freedom (df) = k-1 (where k is the number of response categories). If the null hypothesis is true, the observed and expected frequencies will be close in value and the χ 2 statistic will be close to zero. If the null hypothesis is false, then the χ 2 statistic will be large. Critical values can be found in a table of probabilities for the χ 2 distribution. Here we have df=k-1=3-1=2 and a 5% level of significance. The appropriate critical value is 5.99, and the decision rule is as follows: Reject H 0 if χ 2 > 5.99.
- Step 4. Compute the test statistic.
We now compute the expected frequencies using the sample size and the proportions specified in the null hypothesis. We then substitute the sample data (observed frequencies) and the expected frequencies into the formula for the test statistic identified in Step 2. The computations can be organized as follows.
Notice that the expected frequencies are taken to one decimal place and that the sum of the observed frequencies is equal to the sum of the expected frequencies. The test statistic is computed as follows:
- Step 5. Conclusion.
We reject H 0 because 8.46 > 5.99. We have statistically significant evidence at α=0.05 to show that H 0 is false, or that the distribution of responses is not 0.60, 0.25, 0.15. The p-value is p < 0.005.
In the χ 2 goodness-of-fit test, we conclude that either the distribution specified in H 0 is false (when we reject H 0 ) or that we do not have sufficient evidence to show that the distribution specified in H 0 is false (when we fail to reject H 0 ). Here, we reject H 0 and concluded that the distribution of responses to the exercise question following the implementation of the health promotion campaign was not the same as the distribution prior. The test itself does not provide details of how the distribution has shifted. A comparison of the observed and expected frequencies will provide some insight into the shift (when the null hypothesis is rejected). Does it appear that the health promotion campaign was effective?
Consider the following:
If the null hypothesis were true (i.e., no change from the prior year) we would have expected more students to fall in the "No Regular Exercise" category and fewer in the "Regular Exercise" categories. In the sample, 255/470 = 54% reported no regular exercise and 90/470=19% reported regular exercise. Thus, there is a shift toward more regular exercise following the implementation of the health promotion campaign. There is evidence of a statistical difference, is this a meaningful difference? Is there room for improvement?
The National Center for Health Statistics (NCHS) provided data on the distribution of weight (in categories) among Americans in 2002. The distribution was based on specific values of body mass index (BMI) computed as weight in kilograms over height in meters squared. Underweight was defined as BMI< 18.5, Normal weight as BMI between 18.5 and 24.9, overweight as BMI between 25 and 29.9 and obese as BMI of 30 or greater. Americans in 2002 were distributed as follows: 2% Underweight, 39% Normal Weight, 36% Overweight, and 23% Obese. Suppose we want to assess whether the distribution of BMI is different in the Framingham Offspring sample. Using data from the n=3,326 participants who attended the seventh examination of the Offspring in the Framingham Heart Study we created the BMI categories as defined and observed the following:
- Step 1. Set up hypotheses and determine level of significance.
H 0 : p 1 =0.02, p 2 =0.39, p 3 =0.36, p 4 =0.23 or equivalently
H 0 : Distribution of responses is 0.02, 0.39, 0.36, 0.23
H 1 : H 0 is false. α=0.05
The formula for the test statistic is:
We must assess whether the sample size is adequate. Specifically, we need to check min(np 0 , np 1, ..., n p k ) > 5. The sample size here is n=3,326 and the proportions specified in the null hypothesis are 0.02, 0.39, 0.36 and 0.23. Thus, min( 3326(0.02), 3326(0.39), 3326(0.36), 3326(0.23))=min(66.5, 1297.1, 1197.4, 765.0)=66.5. The sample size is more than adequate, so the formula can be used.
Here we have df=k-1=4-1=3 and a 5% level of significance. The appropriate critical value is 7.81 and the decision rule is as follows: Reject H 0 if χ 2 > 7.81.
We now compute the expected frequencies using the sample size and the proportions specified in the null hypothesis. We then substitute the sample data (observed frequencies) into the formula for the test statistic identified in Step 2. We organize the computations in the following table.
The test statistic is computed as follows:
We reject H 0 because 233.53 > 7.81. We have statistically significant evidence at α=0.05 to show that H 0 is false or that the distribution of BMI in Framingham is different from the national data reported in 2002, p < 0.005.
Again, the χ 2 goodness-of-fit test allows us to assess whether the distribution of responses "fits" a specified distribution. Here we show that the distribution of BMI in the Framingham Offspring Study is different from the national distribution. To understand the nature of the difference we can compare observed and expected frequencies or observed and expected proportions (or percentages). The frequencies are large because of the large sample size, the observed percentages of patients in the Framingham sample are as follows: 0.6% underweight, 28% normal weight, 41% overweight and 30% obese. In the Framingham Offspring sample there are higher percentages of overweight and obese persons (41% and 30% in Framingham as compared to 36% and 23% in the national data), and lower proportions of underweight and normal weight persons (0.6% and 28% in Framingham as compared to 2% and 39% in the national data). Are these meaningful differences?
In the module on hypothesis testing for means and proportions, we discussed hypothesis testing applications with a dichotomous outcome variable in a single population. We presented a test using a test statistic Z to test whether an observed (sample) proportion differed significantly from a historical or external comparator. The chi-square goodness-of-fit test can also be used with a dichotomous outcome and the results are mathematically equivalent.
In the prior module, we considered the following example. Here we show the equivalence to the chi-square goodness-of-fit test.
The NCHS report indicated that in 2002, 75% of children aged 2 to 17 saw a dentist in the past year. An investigator wants to assess whether use of dental services is similar in children living in the city of Boston. A sample of 125 children aged 2 to 17 living in Boston are surveyed and 64 reported seeing a dentist over the past 12 months. Is there a significant difference in use of dental services between children living in Boston and the national data?
We presented the following approach to the test using a Z statistic.
- Step 1. Set up hypotheses and determine level of significance
H 0 : p = 0.75
H 1 : p ≠ 0.75 α=0.05
We must first check that the sample size is adequate. Specifically, we need to check min(np 0 , n(1-p 0 )) = min( 125(0.75), 125(1-0.75))=min(94, 31)=31. The sample size is more than adequate so the following formula can be used
This is a two-tailed test, using a Z statistic and a 5% level of significance. Reject H 0 if Z < -1.960 or if Z > 1.960.
We now substitute the sample data into the formula for the test statistic identified in Step 2. The sample proportion is:

We reject H 0 because -6.15 < -1.960. We have statistically significant evidence at a =0.05 to show that there is a statistically significant difference in the use of dental service by children living in Boston as compared to the national data. (p < 0.0001).
We now conduct the same test using the chi-square goodness-of-fit test. First, we summarize our sample data as follows:
H 0 : p 1 =0.75, p 2 =0.25 or equivalently H 0 : Distribution of responses is 0.75, 0.25
We must assess whether the sample size is adequate. Specifically, we need to check min(np 0 , np 1, ...,np k >) > 5. The sample size here is n=125 and the proportions specified in the null hypothesis are 0.75, 0.25. Thus, min( 125(0.75), 125(0.25))=min(93.75, 31.25)=31.25. The sample size is more than adequate so the formula can be used.
Here we have df=k-1=2-1=1 and a 5% level of significance. The appropriate critical value is 3.84, and the decision rule is as follows: Reject H 0 if χ 2 > 3.84. (Note that 1.96 2 = 3.84, where 1.96 was the critical value used in the Z test for proportions shown above.)
(Note that (-6.15) 2 = 37.8, where -6.15 was the value of the Z statistic in the test for proportions shown above.)
We reject H 0 because 37.8 > 3.84. We have statistically significant evidence at α=0.05 to show that there is a statistically significant difference in the use of dental service by children living in Boston as compared to the national data. (p < 0.0001). This is the same conclusion we reached when we conducted the test using the Z test above. With a dichotomous outcome, Z 2 = χ 2 ! In statistics, there are often several approaches that can be used to test hypotheses.
Tests for Two or More Independent Samples, Discrete Outcome
Here we extend that application of the chi-square test to the case with two or more independent comparison groups. Specifically, the outcome of interest is discrete with two or more responses and the responses can be ordered or unordered (i.e., the outcome can be dichotomous, ordinal or categorical). We now consider the situation where there are two or more independent comparison groups and the goal of the analysis is to compare the distribution of responses to the discrete outcome variable among several independent comparison groups.
The test is called the χ 2 test of independence and the null hypothesis is that there is no difference in the distribution of responses to the outcome across comparison groups. This is often stated as follows: The outcome variable and the grouping variable (e.g., the comparison treatments or comparison groups) are independent (hence the name of the test). Independence here implies homogeneity in the distribution of the outcome among comparison groups.
The null hypothesis in the χ 2 test of independence is often stated in words as: H 0 : The distribution of the outcome is independent of the groups. The alternative or research hypothesis is that there is a difference in the distribution of responses to the outcome variable among the comparison groups (i.e., that the distribution of responses "depends" on the group). In order to test the hypothesis, we measure the discrete outcome variable in each participant in each comparison group. The data of interest are the observed frequencies (or number of participants in each response category in each group). The formula for the test statistic for the χ 2 test of independence is given below.
Test Statistic for Testing H 0 : Distribution of outcome is independent of groups
and we find the critical value in a table of probabilities for the chi-square distribution with df=(r-1)*(c-1).
Here O = observed frequency, E=expected frequency in each of the response categories in each group, r = the number of rows in the two-way table and c = the number of columns in the two-way table. r and c correspond to the number of comparison groups and the number of response options in the outcome (see below for more details). The observed frequencies are the sample data and the expected frequencies are computed as described below. The test statistic is appropriate for large samples, defined as expected frequencies of at least 5 in each of the response categories in each group.
The data for the χ 2 test of independence are organized in a two-way table. The outcome and grouping variable are shown in the rows and columns of the table. The sample table below illustrates the data layout. The table entries (blank below) are the numbers of participants in each group responding to each response category of the outcome variable.
Table - Possible outcomes are are listed in the columns; The groups being compared are listed in rows.
In the table above, the grouping variable is shown in the rows of the table; r denotes the number of independent groups. The outcome variable is shown in the columns of the table; c denotes the number of response options in the outcome variable. Each combination of a row (group) and column (response) is called a cell of the table. The table has r*c cells and is sometimes called an r x c ("r by c") table. For example, if there are 4 groups and 5 categories in the outcome variable, the data are organized in a 4 X 5 table. The row and column totals are shown along the right-hand margin and the bottom of the table, respectively. The total sample size, N, can be computed by summing the row totals or the column totals. Similar to ANOVA, N does not refer to a population size here but rather to the total sample size in the analysis. The sample data can be organized into a table like the above. The numbers of participants within each group who select each response option are shown in the cells of the table and these are the observed frequencies used in the test statistic.
The test statistic for the χ 2 test of independence involves comparing observed (sample data) and expected frequencies in each cell of the table. The expected frequencies are computed assuming that the null hypothesis is true. The null hypothesis states that the two variables (the grouping variable and the outcome) are independent. The definition of independence is as follows:
Two events, A and B, are independent if P(A|B) = P(A), or equivalently, if P(A and B) = P(A) P(B).
The second statement indicates that if two events, A and B, are independent then the probability of their intersection can be computed by multiplying the probability of each individual event. To conduct the χ 2 test of independence, we need to compute expected frequencies in each cell of the table. Expected frequencies are computed by assuming that the grouping variable and outcome are independent (i.e., under the null hypothesis). Thus, if the null hypothesis is true, using the definition of independence:
P(Group 1 and Response Option 1) = P(Group 1) P(Response Option 1).
The above states that the probability that an individual is in Group 1 and their outcome is Response Option 1 is computed by multiplying the probability that person is in Group 1 by the probability that a person is in Response Option 1. To conduct the χ 2 test of independence, we need expected frequencies and not expected probabilities . To convert the above probability to a frequency, we multiply by N. Consider the following small example.
The data shown above are measured in a sample of size N=150. The frequencies in the cells of the table are the observed frequencies. If Group and Response are independent, then we can compute the probability that a person in the sample is in Group 1 and Response category 1 using:
P(Group 1 and Response 1) = P(Group 1) P(Response 1),
P(Group 1 and Response 1) = (25/150) (62/150) = 0.069.
Thus if Group and Response are independent we would expect 6.9% of the sample to be in the top left cell of the table (Group 1 and Response 1). The expected frequency is 150(0.069) = 10.4. We could do the same for Group 2 and Response 1:
P(Group 2 and Response 1) = P(Group 2) P(Response 1),
P(Group 2 and Response 1) = (50/150) (62/150) = 0.138.
The expected frequency in Group 2 and Response 1 is 150(0.138) = 20.7.
Thus, the formula for determining the expected cell frequencies in the χ 2 test of independence is as follows:
Expected Cell Frequency = (Row Total * Column Total)/N.
The above computes the expected frequency in one step rather than computing the expected probability first and then converting to a frequency.
In a prior example we evaluated data from a survey of university graduates which assessed, among other things, how frequently they exercised. The survey was completed by 470 graduates. In the prior example we used the χ 2 goodness-of-fit test to assess whether there was a shift in the distribution of responses to the exercise question following the implementation of a health promotion campaign on campus. We specifically considered one sample (all students) and compared the observed distribution to the distribution of responses the prior year (a historical control). Suppose we now wish to assess whether there is a relationship between exercise on campus and students' living arrangements. As part of the same survey, graduates were asked where they lived their senior year. The response options were dormitory, on-campus apartment, off-campus apartment, and at home (i.e., commuted to and from the university). The data are shown below.
Based on the data, is there a relationship between exercise and student's living arrangement? Do you think where a person lives affect their exercise status? Here we have four independent comparison groups (living arrangement) and a discrete (ordinal) outcome variable with three response options. We specifically want to test whether living arrangement and exercise are independent. We will run the test using the five-step approach.
H 0 : Living arrangement and exercise are independent
H 1 : H 0 is false. α=0.05
The null and research hypotheses are written in words rather than in symbols. The research hypothesis is that the grouping variable (living arrangement) and the outcome variable (exercise) are dependent or related.
- Step 2. Select the appropriate test statistic.
The condition for appropriate use of the above test statistic is that each expected frequency is at least 5. In Step 4 we will compute the expected frequencies and we will ensure that the condition is met.
The decision rule depends on the level of significance and the degrees of freedom, defined as df = (r-1)(c-1), where r and c are the numbers of rows and columns in the two-way data table. The row variable is the living arrangement and there are 4 arrangements considered, thus r=4. The column variable is exercise and 3 responses are considered, thus c=3. For this test, df=(4-1)(3-1)=3(2)=6. Again, with χ 2 tests there are no upper, lower or two-tailed tests. If the null hypothesis is true, the observed and expected frequencies will be close in value and the χ 2 statistic will be close to zero. If the null hypothesis is false, then the χ 2 statistic will be large. The rejection region for the χ 2 test of independence is always in the upper (right-hand) tail of the distribution. For df=6 and a 5% level of significance, the appropriate critical value is 12.59 and the decision rule is as follows: Reject H 0 if c 2 > 12.59.
We now compute the expected frequencies using the formula,
Expected Frequency = (Row Total * Column Total)/N.
The computations can be organized in a two-way table. The top number in each cell of the table is the observed frequency and the bottom number is the expected frequency. The expected frequencies are shown in parentheses.
Notice that the expected frequencies are taken to one decimal place and that the sums of the observed frequencies are equal to the sums of the expected frequencies in each row and column of the table.
Recall in Step 2 a condition for the appropriate use of the test statistic was that each expected frequency is at least 5. This is true for this sample (the smallest expected frequency is 9.6) and therefore it is appropriate to use the test statistic.
We reject H 0 because 60.5 > 12.59. We have statistically significant evidence at a =0.05 to show that H 0 is false or that living arrangement and exercise are not independent (i.e., they are dependent or related), p < 0.005.
Again, the χ 2 test of independence is used to test whether the distribution of the outcome variable is similar across the comparison groups. Here we rejected H 0 and concluded that the distribution of exercise is not independent of living arrangement, or that there is a relationship between living arrangement and exercise. The test provides an overall assessment of statistical significance. When the null hypothesis is rejected, it is important to review the sample data to understand the nature of the relationship. Consider again the sample data.
Because there are different numbers of students in each living situation, it makes the comparisons of exercise patterns difficult on the basis of the frequencies alone. The following table displays the percentages of students in each exercise category by living arrangement. The percentages sum to 100% in each row of the table. For comparison purposes, percentages are also shown for the total sample along the bottom row of the table.
From the above, it is clear that higher percentages of students living in dormitories and in on-campus apartments reported regular exercise (31% and 23%) as compared to students living in off-campus apartments and at home (10% each).
Test Yourself
Pancreaticoduodenectomy (PD) is a procedure that is associated with considerable morbidity. A study was recently conducted on 553 patients who had a successful PD between January 2000 and December 2010 to determine whether their Surgical Apgar Score (SAS) is related to 30-day perioperative morbidity and mortality. The table below gives the number of patients experiencing no, minor, or major morbidity by SAS category.
Question: What would be an appropriate statistical test to examine whether there is an association between Surgical Apgar Score and patient outcome? Using 14.13 as the value of the test statistic for these data, carry out the appropriate test at a 5% level of significance. Show all parts of your test.
In the module on hypothesis testing for means and proportions, we discussed hypothesis testing applications with a dichotomous outcome variable and two independent comparison groups. We presented a test using a test statistic Z to test for equality of independent proportions. The chi-square test of independence can also be used with a dichotomous outcome and the results are mathematically equivalent.
In the prior module, we considered the following example. Here we show the equivalence to the chi-square test of independence.
A randomized trial is designed to evaluate the effectiveness of a newly developed pain reliever designed to reduce pain in patients following joint replacement surgery. The trial compares the new pain reliever to the pain reliever currently in use (called the standard of care). A total of 100 patients undergoing joint replacement surgery agreed to participate in the trial. Patients were randomly assigned to receive either the new pain reliever or the standard pain reliever following surgery and were blind to the treatment assignment. Before receiving the assigned treatment, patients were asked to rate their pain on a scale of 0-10 with higher scores indicative of more pain. Each patient was then given the assigned treatment and after 30 minutes was again asked to rate their pain on the same scale. The primary outcome was a reduction in pain of 3 or more scale points (defined by clinicians as a clinically meaningful reduction). The following data were observed in the trial.
We tested whether there was a significant difference in the proportions of patients reporting a meaningful reduction (i.e., a reduction of 3 or more scale points) using a Z statistic, as follows.
H 0 : p 1 = p 2
H 1 : p 1 ≠ p 2 α=0.05
Here the new or experimental pain reliever is group 1 and the standard pain reliever is group 2.
We must first check that the sample size is adequate. Specifically, we need to ensure that we have at least 5 successes and 5 failures in each comparison group or that:
In this example, we have
Therefore, the sample size is adequate, so the following formula can be used:
Reject H 0 if Z < -1.960 or if Z > 1.960.
We now substitute the sample data into the formula for the test statistic identified in Step 2. We first compute the overall proportion of successes:
We now substitute to compute the test statistic.
- Step 5. Conclusion.
We now conduct the same test using the chi-square test of independence.
H 0 : Treatment and outcome (meaningful reduction in pain) are independent
H 1 : H 0 is false. α=0.05
The formula for the test statistic is:
For this test, df=(2-1)(2-1)=1. At a 5% level of significance, the appropriate critical value is 3.84 and the decision rule is as follows: Reject H0 if χ 2 > 3.84. (Note that 1.96 2 = 3.84, where 1.96 was the critical value used in the Z test for proportions shown above.)
We now compute the expected frequencies using:
The computations can be organized in a two-way table. The top number in each cell of the table is the observed frequency and the bottom number is the expected frequency. The expected frequencies are shown in parentheses.
A condition for the appropriate use of the test statistic was that each expected frequency is at least 5. This is true for this sample (the smallest expected frequency is 22.0) and therefore it is appropriate to use the test statistic.
(Note that (2.53) 2 = 6.4, where 2.53 was the value of the Z statistic in the test for proportions shown above.)
Chi-Squared Tests in R
The video below by Mike Marin demonstrates how to perform chi-squared tests in the R programming language.
Answer to Problem on Pancreaticoduodenectomy and Surgical Apgar Scores
We have 3 independent comparison groups (Surgical Apgar Score) and a categorical outcome variable (morbidity/mortality). We can run a Chi-Squared test of independence.
H 0 : Apgar scores and patient outcome are independent of one another.
H A : Apgar scores and patient outcome are not independent.
Chi-squared = 14.3
Since 14.3 is greater than 9.49, we reject H 0.
There is an association between Apgar scores and patient outcome. The lowest Apgar score group (0 to 4) experienced the highest percentage of major morbidity or mortality (16 out of 57=28%) compared to the other Apgar score groups.

An official website of the United States government
The .gov means it’s official. Federal government websites often end in .gov or .mil. Before sharing sensitive information, make sure you’re on a federal government site.
The site is secure. The https:// ensures that you are connecting to the official website and that any information you provide is encrypted and transmitted securely.
- Publications
- Account settings
Preview improvements coming to the PMC website in October 2024. Learn More or Try it out now .
- Advanced Search
- Journal List
- Biochem Med (Zagreb)
- v.23(2); 2013 Jun
The Chi-square test of independence
The Chi-square statistic is a non-parametric (distribution free) tool designed to analyze group differences when the dependent variable is measured at a nominal level. Like all non-parametric statistics, the Chi-square is robust with respect to the distribution of the data. Specifically, it does not require equality of variances among the study groups or homoscedasticity in the data. It permits evaluation of both dichotomous independent variables, and of multiple group studies. Unlike many other non-parametric and some parametric statistics, the calculations needed to compute the Chi-square provide considerable information about how each of the groups performed in the study. This richness of detail allows the researcher to understand the results and thus to derive more detailed information from this statistic than from many others.
The Chi-square is a significance statistic, and should be followed with a strength statistic. The Cramer’s V is the most common strength test used to test the data when a significant Chi-square result has been obtained. Advantages of the Chi-square include its robustness with respect to distribution of the data, its ease of computation, the detailed information that can be derived from the test, its use in studies for which parametric assumptions cannot be met, and its flexibility in handling data from both two group and multiple group studies. Limitations include its sample size requirements, difficulty of interpretation when there are large numbers of categories (20 or more) in the independent or dependent variables, and tendency of the Cramer’s V to produce relative low correlation measures, even for highly significant results.
Introduction
The Chi-square test of independence (also known as the Pearson Chi-square test, or simply the Chi-square) is one of the most useful statistics for testing hypotheses when the variables are nominal, as often happens in clinical research. Unlike most statistics, the Chi-square (χ 2 ) can provide information not only on the significance of any observed differences, but also provides detailed information on exactly which categories account for any differences found. Thus, the amount and detail of information this statistic can provide renders it one of the most useful tools in the researcher’s array of available analysis tools. As with any statistic, there are requirements for its appropriate use, which are called “assumptions” of the statistic. Additionally, the χ 2 is a significance test, and should always be coupled with an appropriate test of strength.
The Chi-square test is a non-parametric statistic, also called a distribution free test. Non-parametric tests should be used when any one of the following conditions pertains to the data:
- The level of measurement of all the variables is nominal or ordinal.
- The sample sizes of the study groups are unequal; for the χ 2 the groups may be of equal size or unequal size whereas some parametric tests require groups of equal or approximately equal size.
- The distribution of the data was seriously skewed or kurtotic (parametric tests assume approximately normal distribution of the dependent variable), and thus the researcher must use a distribution free statistic rather than a parametric statistic.
- The data violate the assumptions of equal variance or homoscedasticity.
- For any of a number of reasons ( 1 ), the continuous data were collapsed into a small number of categories, and thus the data are no longer interval or ratio.
Assumptions of the Chi-square
As with parametric tests, the non-parametric tests, including the χ 2 assume the data were obtained through random selection. However, it is not uncommon to find inferential statistics used when data are from convenience samples rather than random samples. (To have confidence in the results when the random sampling assumption is violated, several replication studies should be performed with essentially the same result obtained). Each non-parametric test has its own specific assumptions as well. The assumptions of the Chi-square include:
- The data in the cells should be frequencies, or counts of cases rather than percentages or some other transformation of the data.
- The levels (or categories) of the variables are mutually exclusive. That is, a particular subject fits into one and only one level of each of the variables.
- Each subject may contribute data to one and only one cell in the χ 2 . If, for example, the same subjects are tested over time such that the comparisons are of the same subjects at Time 1, Time 2, Time 3, etc., then χ 2 may not be used.
- The study groups must be independent. This means that a different test must be used if the two groups are related. For example, a different test must be used if the researcher’s data consists of paired samples, such as in studies in which a parent is paired with his or her child.
- There are 2 variables, and both are measured as categories, usually at the nominal level. However, data may be ordinal data. Interval or ratio data that have been collapsed into ordinal categories may also be used. While Chi-square has no rule about limiting the number of cells (by limiting the number of categories for each variable), a very large number of cells (over 20) can make it difficult to meet assumption #6 below, and to interpret the meaning of the results.
- The value of the cell expecteds should be 5 or more in at least 80% of the cells, and no cell should have an expected of less than one ( 3 ). This assumption is most likely to be met if the sample size equals at least the number of cells multiplied by 5. Essentially, this assumption specifies the number of cases (sample size) needed to use the χ 2 for any number of cells in that χ 2 . This requirement will be fully explained in the example of the calculation of the statistic in the case study example.
To illustrate the calculation and interpretation of the χ 2 statistic, the following case example will be used:
The owner of a laboratory wants to keep sick leave as low as possible by keeping employees healthy through disease prevention programs. Many employees have contracted pneumonia leading to productivity problems due to sick leave from the disease. There is a vaccine for pneumococcal pneumonia, and the owner believes that it is important to get as many employees vaccinated as possible. Due to a production problem at the company that produces the vaccine, there is only enough vaccine for half the employees. In effect, there are two groups; employees who received the vaccine and employees who did not receive the vaccine. The company sent a nurse to every employee who contracted pneumonia to provide home health care and to take a sputum sample for culture to determine the causative agent. They kept track of the number of employees who contracted pneumonia and which type of pneumonia each had. The data were organized as follows:
- Group 1: Not provided with the vaccine (unvaccinated control group, N = 92)
- Group 2: Provided with the vaccine (vaccinated experimental group, N = 92)
In this case, the independent variable is vaccination status (vaccinated versus unvaccinated). The dependent variable is health outcome with three levels:
- contracted pneumoccal pneumonia;
- contracted another type of pneumonia; and
- did not contract pneumonia.
The company wanted to know if providing the vaccine made a difference. To answer this question, they must choose a statistic that can test for differences when all the variables are nominal. The χ 2 statistic was used to test the question, “Was there a difference in incidence of pneumonia between the two groups?” At the end of the winter, Table 1 was constructed to illustrate the occurrence of pneumonia among the employees.
Results of the vaccination program.
Calculating Chi-square
With the data in table form, the researcher can proceed with calculating the χ 2 statistic to find out if the vaccination program made any difference in the health outcomes of the employees. The formula for calculating a Chi-Square is:
The first step in calculating a χ 2 is to calculate the sum of each row, and the sum of each column. These sums are called the “marginals” and there are row marginal values and column marginal values. The marginal values for the case study data are presented in Table 2 .
Calculation of marginals.
The second step is to calculate the expected values for each cell. In the Chi-square statistic, the “expected” values represent an estimate of how the cases would be distributed if there were NO vaccine effect. Expected values must reflect both the incidence of cases in each category and the unbiased distribution of cases if there is no vaccine effect. This means the statistic cannot just count the total N and divide by 6 for the expected number in each cell. That would not take account of the fact that more subjects stayed healthy regardless of whether they were vaccinated or not. Chi-Square expecteds are calculated as follows:
Specifically, for each cell, its row marginal is multiplied by its column marginal, and that product is divided by the sample size. For Cell 1, the math is as follows: (28 × 92)/184 = 13.92. Table 3 provides the results of this calculation for each cell. Once the expected values have been calculated, the cell χ 2 values are calculated with the following formula:
The cell χ 2 for the first cell in the case study data is calculated as follows: (23−13.93) 2 /13.93 = 5.92. The cell χ 2 value for each cellis the value in parentheses in each of the cells in Table 3 .
Cell expected values and (cell Chi-square values).
Once the cell χ 2 values have been calculated, they are summed to obtain the χ 2 statistic for the table. In this case, the χ 2 is 12.35 (rounded). The Chi-square table requires the table’s degrees of freedom (df) in order to determine the significance level of the statistic. The degrees of freedom for a χ 2 table are calculated with the formula:
For example, a 2 × 2 table has 1 df. (2−1) × (2−1) = 1. A 3 × 3 table has (3−1) × (3−1) = 4 df. A 4 × 5 table has (4−1) × (5−1) = 3 × 4 = 12 df. Assuming a χ 2 value of 12.35 with each of these different df levels (1, 4, and 12), the significance levels from a table of χ 2 values, the significance levels are: df = 1, P < 0.001, df = 4, P < 0.025, and df = 12, P > 0.10. Note, as degrees of freedom increase, the P-level becomes less significant, until the χ 2 value of 12.35 is no longer statistically significant at the 0.05 level, because P was greater than 0.10.
For the sample table with 3 rows and 2 columns, df = (3−1) × (2−1) = 2 × 1 = 2. A Chi-square table of significances is available in many elementary statistics texts and on many Internet sites. Using a χ 2 table, the significance of a Chi-square value of 12.35 with 2 df equals P < 0.005. This value may be rounded to P < 0.01 for convenience. The exact significance when the Chi-square is calculated through a statistical program is found to be P = 0.0011.
As the P-value of the table is less than P < 0.05, the researcher rejects the null hypothesis and accepts the alternate hypothesis: “There is a difference in occurrence of pneumococcal pneumonia between the vaccinated and unvaccinated groups.” However, this result does not specify what that difference might be. To fully interpret the result, it is useful to look at the cell χ 2 values.
Interpreting cell χ 2 values
It can be seen in Table 3 that the largest cell χ 2 value of 5.92 occurs in Cell 1. This is a result of the observed value being 23 while only 13.92 were expected. Therefore, this cell has a much larger number of observed cases than would be expected by chance. Cell 1 reflects the number of unvaccinated employees who contracted pneumococcal pneumonia. This means that the number of unvaccinated people who contracted pneumococcal pneumonia was significantly greater than expected. The second largest cell χ 2 value of 4.56 is located in Cell 2. However, in this cell we discover that the number of observed cases was much lower than expected (Observed = 5, Expected = 12.57). This means that a significantly lower number of vaccinated subjects contracted pneumococcal pneumonia than would be expected if the vaccine had no effect. No other cell has a cell χ 2 value greater than 0.99.
A cell χ 2 value less than 1.0 should be interpreted as the number of observed cases being approximately equal to the number of expected cases, meaning there is no vaccination effect on any of the other cells. In the case study example, all other cells produced cell χ 2 values below 1.0. Therefore the company can conclude that there was no difference between the two groups for incidence of non-pneumococcal pneumonia. It can be seen that for both groups, the majority of employees stayed healthy. The meaningful result was that there were significantly fewer cases of pneumococcal pneumonia among the vaccinated employees and significantly more cases among the unvaccinated employees. As a result, the company should conclude that the vaccination program did reduce the incidence of pneumoccal pneumonia.
Very few statistical programs provide tables of cell expecteds and cell χ 2 values as part of the default output. Some programs will produce those tables as an option, and that option should be used to examine the cell χ 2 values. If the program provides an option to print out only the cell χ 2 value (but not cell expecteds), the direction of the χ 2 value provides information. A positive cell χ 2 value means that the observed value is higher than the expected value, and a negative cell χ 2 value (e.g. −12.45) means the observed cases are less than the expected number of cases. When the program does not provide either option, all the researcher can conclude is this: The overall table provides evidence that the two groups are independent (significantly different because P < 0.05), or are not independent (P > 0.05). Most researchers inspect the table to estimate which cells are overrepresented with a large number of cases versus those which have a small number of cases. However, without access to cell expecteds or cell χ 2 values, the interpretation of the direction of the group differences is less precise. Given the ease of calculating the cell expecteds and χ 2 values, researchers may want to hand calculate those values to enhance interpretation.
Chi-square and closely related tests
One might ask if, in this case, the Chi-square was the best or only test the researcher could have used. Nominal variables require the use of non-parametric tests, and there are three commonly used significance tests that can be used for this type of nominal data. The first and most commonly used is the Chi-square. The second is the Fisher’s exact test, which is a bit more precise than the Chi-square, but it is used only for 2 × 2 Tables ( 4 ). For example, if the only options in the case study were pneumonia versus no pneumonia, the table would have 2 rows and 2 columns and the correct test would be the Fisher’s exact. The case study example requires a 2 × 3 table and thus the data are not suitable for the Fisher’s exact test.
The third test is the maximum likelihood ratio Chi-square test which is most often used when the data set is too small to meet the sample size assumption of the Chi-square test. As exhibited by the table of expected values for the case study, the cell expected requirements of the Chi-square were met by the data in the example. Specifically, there are 6 cells in the table. To meet the requirement that 80% of the cells have expected values of 5 or more, this table must have 6 × 0.8 = 4.8 rounded to 5. This table meets the requirement that at least 5 of the 6 cells must have cell expected of 5 or more, and so there is no need to use the maximum likelihood ratio chi-square. Suppose the sample size were much smaller. Suppose the sample size was smaller and the table had the data in Table 4 .
Example of a table that violates cell expected values.
Sample raw data presented first, sample expected values in parentheses, and cell follow the slash.
Although the total sample size of 39 exceeds the value of 5 cases × 6 cells = 30, the very low distribution of cases in 4 of the cells is of concern. When the cell expecteds are calculated, it can be seen that 4 of the 6 cells have expecteds below 5, and thus this table violates the χ 2 test assumption. This table should be tested with a maximum likelihood ratio Chi-square test.
When researchers use the Chi-square test in violation of one or more assumptions, the result may or may not be reliable. In this author’s experience of having output from both the appropriate and inappropriate tests on the same data, one of three outcomes are possible:
First, the appropriate and the inappropriate test may give the same results.
Second, the appropriate test may produce a significant result while the inappropriate test provides a result that is not statistically significant, which is a Type II error.
Third, the appropriate test may provide a non-significant result while the inappropriate test may provide a significant result, which is a Type I error.
Strength test for the Chi-square
The researcher’s work is not quite done yet. Finding a significant difference merely means that the differences between the vaccinated and unvaccinated groups have less than 1.1 in a thousand chances of being in error (P = 0.0011). That is, there are 1.1 in one thousand chances that there really is no difference between the two groups for contracting pneumococcal pneumonia, and that the researcher made a Type I error. That is a sufficiently remote probability of error that in this case, the company can be confident that the vaccination made a difference. While useful, this is not complete information. It is necessary to know the strength of the association as well as the significance.
Statistical significance does not necessarily imply clinical importance. Clinical significance is usually a function of how much improvement is produced by the treatment. For example, if there was a significant difference, but the vaccine only reduced pneumonias by two cases, it might not be worth the company’s money to vaccinate 184 people (at a cost of $20 per person) to eliminate only two cases. In this case study, the vaccinated group experienced only 5 cases out of 92 employees (a rate of 5%) while the unvaccinated group experienced 23 cases out of 92 employees (a rate of 25%). While it is always a matter of judgment as to whether the results are worth the investment, many employers would view 25% of their workforce becoming ill with a preventable infectious illness as an undesirable outcome. There is, however, a more standardized strength test for the Chi-Square.
Statistical strength tests are correlation measures. For the Chi-square, the most commonly used strength test is the Cramer’s V test. It is easily calculated with the following formula:
Where n is the number of rows or number of columns, whichever is less. For the example, the V is 0.259 or rounded, 0.26 as calculated below.
The Cramer’s V is a form of a correlation and is interpreted exactly the same. For any correlation, a value of 0.26 is a weak correlation. It should be noted that a relatively weak correlation is all that can be expected when a phenomena is only partially dependent on the independent variable.
In the case study, five vaccinated people did contract pneumococcal pneumonia, but vaccinated or not, the majority of employees remained healthy. Clearly, most employees will not get pneumonia. This fact alone makes it difficult to obtain a moderate or high correlation coefficient. The amount of change the treatment (vaccine) can produce is limited by the relatively low rate of disease in the population of employees. While the correlation value is low, it is statistically significant, and the clinical importance of reducing a rate of 25% incidence to 5% incidence of the disease would appear to be clinically worthwhile. These are the factors the researcher should take into account when interpreting this statistical result.
Summary and conclusions
The Chi-square is a valuable analysis tool that provides considerable information about the nature of research data. It is a powerful statistic that enables researchers to test hypotheses about variables measured at the nominal level. As with all inferential statistics, the results are most reliable when the data are collected from randomly selected subjects, and when sample sizes are sufficiently large that they produce appropriate statistical power. The Chi-square is also an excellent tool to use when violations of assumptions of equal variances and homoscedascity are violated and parametric statistics such as the t-test and ANOVA cannot provide reliable results. As the Chi-Square and its strength test, the Cramer’s V are both simple to compute, it is an especially convenient tool for researchers in the field where statistical programs may not be easily accessed. However, most statistical programs provide not only the Chi-square and Cramer’s V, but also a variety of other non-parametric tools for both significance and strength testing.
Potential conflict of interest
None declared.
Teach yourself statistics
Chi-Square Test of Independence
This lesson explains how to conduct a chi-square test for independence . The test is applied when you have two categorical variables from a single population. It is used to determine whether there is a significant association between the two variables.
For example, in an election survey, voters might be classified by gender (male or female) and voting preference (Democrat, Republican, or Independent). We could use a chi-square test for independence to determine whether gender is related to voting preference. The sample problem at the end of the lesson considers this example.
When to Use Chi-Square Test for Independence
The test procedure described in this lesson is appropriate when the following conditions are met:
- The sampling method is simple random sampling .
- The variables under study are each categorical .
- If sample data are displayed in a contingency table , the expected frequency count for each cell of the table is at least 5.
This approach consists of four steps: (1) state the hypotheses, (2) formulate an analysis plan, (3) analyze sample data, and (4) interpret results.
State the Hypotheses
Suppose that Variable A has r levels, and Variable B has c levels. The null hypothesis states that knowing the level of Variable A does not help you predict the level of Variable B. That is, the variables are independent.
H o : Variable A and Variable B are independent.
H a : Variable A and Variable B are not independent.
The alternative hypothesis is that knowing the level of Variable A can help you predict the level of Variable B.
Note: Support for the alternative hypothesis suggests that the variables are related; but the relationship is not necessarily causal, in the sense that one variable "causes" the other.
Formulate an Analysis Plan
The analysis plan describes how to use sample data to accept or reject the null hypothesis. The plan should specify the following elements.
- Significance level. Often, researchers choose significance levels equal to 0.01, 0.05, or 0.10; but any value between 0 and 1 can be used.
- Test method. Use the chi-square test for independence to determine whether there is a significant relationship between two categorical variables.
Analyze Sample Data
Using sample data, find the degrees of freedom, expected frequencies, test statistic, and the P-value associated with the test statistic. The approach described in this section is illustrated in the sample problem at the end of this lesson.
DF = (r - 1) * (c - 1)
E r,c = (n r * n c ) / n
Χ 2 = Σ [ (O r,c - E r,c ) 2 / E r,c ]
- P-value. The P-value is the probability of observing a sample statistic as extreme as the test statistic. Since the test statistic is a chi-square, use the Chi-Square Distribution Calculator to assess the probability associated with the test statistic. Use the degrees of freedom computed above.
Interpret Results
If the sample findings are unlikely, given the null hypothesis, the researcher rejects the null hypothesis. Typically, this involves comparing the P-value to the significance level , and rejecting the null hypothesis when the P-value is less than the significance level.
Test Your Understanding
A public opinion poll surveyed a simple random sample of 1000 voters. Respondents were classified by gender (male or female) and by voting preference (Republican, Democrat, or Independent). Results are shown in the contingency table below.
Is there a gender gap? Do the men's voting preferences differ significantly from the women's preferences? Use a 0.05 level of significance.
The solution to this problem takes four steps: (1) state the hypotheses, (2) formulate an analysis plan, (3) analyze sample data, and (4) interpret results. We work through those steps below:
H o : Gender and voting preferences are independent.
H a : Gender and voting preferences are not independent.
- Formulate an analysis plan . For this analysis, the significance level is 0.05. Using sample data, we will conduct a chi-square test for independence .
DF = (r - 1) * (c - 1) = (2 - 1) * (3 - 1) = 2
E r,c = (n r * n c ) / n E 1,1 = (400 * 450) / 1000 = 180000/1000 = 180 E 1,2 = (400 * 450) / 1000 = 180000/1000 = 180 E 1,3 = (400 * 100) / 1000 = 40000/1000 = 40 E 2,1 = (600 * 450) / 1000 = 270000/1000 = 270 E 2,2 = (600 * 450) / 1000 = 270000/1000 = 270 E 2,3 = (600 * 100) / 1000 = 60000/1000 = 60
Χ 2 = Σ [ (O r,c - E r,c ) 2 / E r,c ] Χ 2 = (200 - 180) 2 /180 + (150 - 180) 2 /180 + (50 - 40) 2 /40 + (250 - 270) 2 /270 + (300 - 270) 2 /270 + (50 - 60) 2 /60 Χ 2 = 400/180 + 900/180 + 100/40 + 400/270 + 900/270 + 100/60 Χ 2 = 2.22 + 5.00 + 2.50 + 1.48 + 3.33 + 1.67 = 16.2
where DF is the degrees of freedom, r is the number of levels of gender, c is the number of levels of the voting preference, n r is the number of observations from level r of gender, n c is the number of observations from level c of voting preference, n is the number of observations in the sample, E r,c is the expected frequency count when gender is level r and voting preference is level c , and O r,c is the observed frequency count when gender is level r voting preference is level c .
The P-value is the probability that a chi-square statistic having 2 degrees of freedom is more extreme than 16.2. We use the Chi-Square Distribution Calculator to find P(Χ 2 > 16.2) = 0.0003.
- Interpret results . Since the P-value (0.0003) is less than the significance level (0.05), we cannot accept the null hypothesis. Thus, we conclude that there is a relationship between gender and voting preference.
Note: If you use this approach on an exam, you may also want to mention why this approach is appropriate. Specifically, the approach is appropriate because the sampling method was simple random sampling, the variables under study were categorical, and the expected frequency count was at least 5 in each cell of the contingency table.
- Flashes Safe Seven
- FlashLine Login
- Faculty & Staff Phone Directory
- Emeriti or Retiree
- All Departments
- Maps & Directions
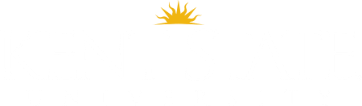
- Building Guide
- Departments
- Directions & Parking
- Faculty & Staff
- Give to University Libraries
- Library Instructional Spaces
- Mission & Vision
- Newsletters
- Circulation
- Course Reserves / Core Textbooks
- Equipment for Checkout
- Interlibrary Loan
- Library Instruction
- Library Tutorials
- My Library Account
- Open Access Kent State
- Research Support Services
- Statistical Consulting
- Student Multimedia Studio
- Citation Tools
- Databases A-to-Z
- Databases By Subject
- Digital Collections
- Discovery@Kent State
- Government Information
- Journal Finder
- Library Guides
- Connect from Off-Campus
- Library Workshops
- Subject Librarians Directory
- Suggestions/Feedback
- Writing Commons
- Academic Integrity
- Jobs for Students
- International Students
- Meet with a Librarian
- Study Spaces
- University Libraries Student Scholarship
- Affordable Course Materials
- Copyright Services
- Selection Manager
- Suggest a Purchase
Library Locations at the Kent Campus
- Architecture Library
- Fashion Library
- Map Library
- Performing Arts Library
- Special Collections and Archives
Regional Campus Libraries
- East Liverpool
- College of Podiatric Medicine

- Kent State University
- SPSS Tutorials
Chi-Square Test of Independence
Spss tutorials: chi-square test of independence.
- The SPSS Environment
- The Data View Window
- Using SPSS Syntax
- Data Creation in SPSS
- Importing Data into SPSS
- Variable Types
- Date-Time Variables in SPSS
- Defining Variables
- Creating a Codebook
- Computing Variables
- Computing Variables: Mean Centering
- Computing Variables: Recoding Categorical Variables
- Computing Variables: Recoding String Variables into Coded Categories (Automatic Recode)
- rank transform converts a set of data values by ordering them from smallest to largest, and then assigning a rank to each value. In SPSS, the Rank Cases procedure can be used to compute the rank transform of a variable." href="https://libguides.library.kent.edu/SPSS/RankCases" style="" >Computing Variables: Rank Transforms (Rank Cases)
- Weighting Cases
- Sorting Data
- Grouping Data
- Descriptive Stats for One Numeric Variable (Explore)
- Descriptive Stats for One Numeric Variable (Frequencies)
- Descriptive Stats for Many Numeric Variables (Descriptives)
- Descriptive Stats by Group (Compare Means)
- Frequency Tables
- Working with "Check All That Apply" Survey Data (Multiple Response Sets)
- Pearson Correlation
- One Sample t Test
- Paired Samples t Test
- Independent Samples t Test
- One-Way ANOVA
- How to Cite the Tutorials
Sample Data Files
Our tutorials reference a dataset called "sample" in many examples. If you'd like to download the sample dataset to work through the examples, choose one of the files below:
- Data definitions (*.pdf)
- Data - Comma delimited (*.csv)
- Data - Tab delimited (*.txt)
- Data - Excel format (*.xlsx)
- Data - SAS format (*.sas7bdat)
- Data - SPSS format (*.sav)
- SPSS Syntax (*.sps) Syntax to add variable labels, value labels, set variable types, and compute several recoded variables used in later tutorials.
- SAS Syntax (*.sas) Syntax to read the CSV-format sample data and set variable labels and formats/value labels.
The Chi-Square Test of Independence determines whether there is an association between categorical variables (i.e., whether the variables are independent or related). It is a nonparametric test.
This test is also known as:
- Chi-Square Test of Association.
This test utilizes a contingency table to analyze the data. A contingency table (also known as a cross-tabulation , crosstab , or two-way table ) is an arrangement in which data is classified according to two categorical variables. The categories for one variable appear in the rows, and the categories for the other variable appear in columns. Each variable must have two or more categories. Each cell reflects the total count of cases for a specific pair of categories.
There are several tests that go by the name "chi-square test" in addition to the Chi-Square Test of Independence. Look for context clues in the data and research question to make sure what form of the chi-square test is being used.
Common Uses
The Chi-Square Test of Independence is commonly used to test the following:
- Statistical independence or association between two categorical variables.
The Chi-Square Test of Independence can only compare categorical variables. It cannot make comparisons between continuous variables or between categorical and continuous variables. Additionally, the Chi-Square Test of Independence only assesses associations between categorical variables, and can not provide any inferences about causation.
If your categorical variables represent "pre-test" and "post-test" observations, then the chi-square test of independence is not appropriate . This is because the assumption of the independence of observations is violated. In this situation, McNemar's Test is appropriate.
Data Requirements
Your data must meet the following requirements:
- Two categorical variables.
- Two or more categories (groups) for each variable.
- There is no relationship between the subjects in each group.
- The categorical variables are not "paired" in any way (e.g. pre-test/post-test observations).
- Expected frequencies for each cell are at least 1.
- Expected frequencies should be at least 5 for the majority (80%) of the cells.
The null hypothesis ( H 0 ) and alternative hypothesis ( H 1 ) of the Chi-Square Test of Independence can be expressed in two different but equivalent ways:
H 0 : "[ Variable 1 ] is independent of [ Variable 2 ]" H 1 : "[ Variable 1 ] is not independent of [ Variable 2 ]"
H 0 : "[ Variable 1 ] is not associated with [ Variable 2 ]" H 1 : "[ Variable 1 ] is associated with [ Variable 2 ]"
Test Statistic
The test statistic for the Chi-Square Test of Independence is denoted Χ 2 , and is computed as:
$$ \chi^{2} = \sum_{i=1}^{R}{\sum_{j=1}^{C}{\frac{(o_{ij} - e_{ij})^{2}}{e_{ij}}}} $$
\(o_{ij}\) is the observed cell count in the i th row and j th column of the table
\(e_{ij}\) is the expected cell count in the i th row and j th column of the table, computed as
$$ e_{ij} = \frac{\mathrm{ \textrm{row } \mathit{i}} \textrm{ total} * \mathrm{\textrm{col } \mathit{j}} \textrm{ total}}{\textrm{grand total}} $$
The quantity ( o ij - e ij ) is sometimes referred to as the residual of cell ( i , j ), denoted \(r_{ij}\).
The calculated Χ 2 value is then compared to the critical value from the Χ 2 distribution table with degrees of freedom df = ( R - 1)( C - 1) and chosen confidence level. If the calculated Χ 2 value > critical Χ 2 value, then we reject the null hypothesis.
Data Set-Up
There are two different ways in which your data may be set up initially. The format of the data will determine how to proceed with running the Chi-Square Test of Independence. At minimum, your data should include two categorical variables (represented in columns) that will be used in the analysis. The categorical variables must include at least two groups. Your data may be formatted in either of the following ways:
If you have the raw data (each row is a subject):
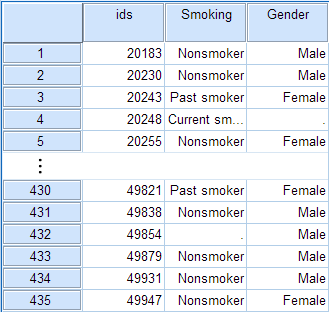
- Cases represent subjects, and each subject appears once in the dataset. That is, each row represents an observation from a unique subject.
- The dataset contains at least two nominal categorical variables (string or numeric). The categorical variables used in the test must have two or more categories.
If you have frequencies (each row is a combination of factors):
An example of using the chi-square test for this type of data can be found in the Weighting Cases tutorial .

- Each row in the dataset represents a distinct combination of the categories.
- The value in the "frequency" column for a given row is the number of unique subjects with that combination of categories.
- You should have three variables: one representing each category, and a third representing the number of occurrences of that particular combination of factors.
- Before running the test, you must activate Weight Cases, and set the frequency variable as the weight.
Run a Chi-Square Test of Independence
In SPSS, the Chi-Square Test of Independence is an option within the Crosstabs procedure. Recall that the Crosstabs procedure creates a contingency table or two-way table , which summarizes the distribution of two categorical variables.
To create a crosstab and perform a chi-square test of independence, click Analyze > Descriptive Statistics > Crosstabs .

A Row(s): One or more variables to use in the rows of the crosstab(s). You must enter at least one Row variable.
B Column(s): One or more variables to use in the columns of the crosstab(s). You must enter at least one Column variable.
Also note that if you specify one row variable and two or more column variables, SPSS will print crosstabs for each pairing of the row variable with the column variables. The same is true if you have one column variable and two or more row variables, or if you have multiple row and column variables. A chi-square test will be produced for each table. Additionally, if you include a layer variable, chi-square tests will be run for each pair of row and column variables within each level of the layer variable.
C Layer: An optional "stratification" variable. If you have turned on the chi-square test results and have specified a layer variable, SPSS will subset the data with respect to the categories of the layer variable, then run chi-square tests between the row and column variables. (This is not equivalent to testing for a three-way association, or testing for an association between the row and column variable after controlling for the layer variable.)
D Statistics: Opens the Crosstabs: Statistics window, which contains fifteen different inferential statistics for comparing categorical variables.

To run the Chi-Square Test of Independence, make sure that the Chi-square box is checked.
E Cells: Opens the Crosstabs: Cell Display window, which controls which output is displayed in each cell of the crosstab. (Note: in a crosstab, the cells are the inner sections of the table. They show the number of observations for a given combination of the row and column categories.) There are three options in this window that are useful (but optional) when performing a Chi-Square Test of Independence:

1 Observed : The actual number of observations for a given cell. This option is enabled by default.
2 Expected : The expected number of observations for that cell (see the test statistic formula).
3 Unstandardized Residuals : The "residual" value, computed as observed minus expected.
F Format: Opens the Crosstabs: Table Format window, which specifies how the rows of the table are sorted.

Example: Chi-square Test for 3x2 Table
Problem statement.
In the sample dataset, respondents were asked their gender and whether or not they were a cigarette smoker. There were three answer choices: Nonsmoker, Past smoker, and Current smoker. Suppose we want to test for an association between smoking behavior (nonsmoker, current smoker, or past smoker) and gender (male or female) using a Chi-Square Test of Independence (we'll use α = 0.05).
Before the Test
Before we test for "association", it is helpful to understand what an "association" and a "lack of association" between two categorical variables looks like. One way to visualize this is using clustered bar charts. Let's look at the clustered bar chart produced by the Crosstabs procedure.
This is the chart that is produced if you use Smoking as the row variable and Gender as the column variable (running the syntax later in this example):
The "clusters" in a clustered bar chart are determined by the row variable (in this case, the smoking categories). The color of the bars is determined by the column variable (in this case, gender). The height of each bar represents the total number of observations in that particular combination of categories.
This type of chart emphasizes the differences within the categories of the row variable. Notice how within each smoking category, the heights of the bars (i.e., the number of males and females) are very similar. That is, there are an approximately equal number of male and female nonsmokers; approximately equal number of male and female past smokers; approximately equal number of male and female current smokers. If there were an association between gender and smoking, we would expect these counts to differ between groups in some way.
Running the Test
- Open the Crosstabs dialog ( Analyze > Descriptive Statistics > Crosstabs ).
- Select Smoking as the row variable, and Gender as the column variable.
- Click Statistics . Check Chi-square , then click Continue .
- (Optional) Check the box for Display clustered bar charts .
The first table is the Case Processing summary, which tells us the number of valid cases used for analysis. Only cases with nonmissing values for both smoking behavior and gender can be used in the test.
The next tables are the crosstabulation and chi-square test results.
The key result in the Chi-Square Tests table is the Pearson Chi-Square.
- The value of the test statistic is 3.171.
- The footnote for this statistic pertains to the expected cell count assumption (i.e., expected cell counts are all greater than 5): no cells had an expected count less than 5, so this assumption was met.
- Because the test statistic is based on a 3x2 crosstabulation table, the degrees of freedom (df) for the test statistic is $$ df = (R - 1)*(C - 1) = (3 - 1)*(2 - 1) = 2*1 = 2 $$.
- The corresponding p-value of the test statistic is p = 0.205.
Decision and Conclusions
Since the p-value is greater than our chosen significance level ( α = 0.05), we do not reject the null hypothesis. Rather, we conclude that there is not enough evidence to suggest an association between gender and smoking.
Based on the results, we can state the following:
- No association was found between gender and smoking behavior ( Χ 2 (2)> = 3.171, p = 0.205).

Example: Chi-square Test for 2x2 Table
Let's continue the row and column percentage example from the Crosstabs tutorial, which described the relationship between the variables RankUpperUnder (upperclassman/underclassman) and LivesOnCampus (lives on campus/lives off-campus). Recall that the column percentages of the crosstab appeared to indicate that upperclassmen were less likely than underclassmen to live on campus:
- The proportion of underclassmen who live off campus is 34.8%, or 79/227.
- The proportion of underclassmen who live on campus is 65.2%, or 148/227.
- The proportion of upperclassmen who live off campus is 94.4%, or 152/161.
- The proportion of upperclassmen who live on campus is 5.6%, or 9/161.
Suppose that we want to test the association between class rank and living on campus using a Chi-Square Test of Independence (using α = 0.05).
The clustered bar chart from the Crosstabs procedure can act as a complement to the column percentages above. Let's look at the chart produced by the Crosstabs procedure for this example:
The height of each bar represents the total number of observations in that particular combination of categories. The "clusters" are formed by the row variable (in this case, class rank). This type of chart emphasizes the differences within the underclassmen and upperclassmen groups. Here, the differences in number of students living on campus versus living off-campus is much starker within the class rank groups.
- Select RankUpperUnder as the row variable, and LiveOnCampus as the column variable.
- (Optional) Click Cells . Under Counts, check the boxes for Observed and Expected , and under Residuals, click Unstandardized . Then click Continue .
The first table is the Case Processing summary, which tells us the number of valid cases used for analysis. Only cases with nonmissing values for both class rank and living on campus can be used in the test.
The next table is the crosstabulation. If you elected to check off the boxes for Observed Count, Expected Count, and Unstandardized Residuals, you should see the following table:
With the Expected Count values shown, we can confirm that all cells have an expected value greater than 5.
These numbers can be plugged into the chi-square test statistic formula:
$$ \chi^{2} = \sum_{i=1}^{R}{\sum_{j=1}^{C}{\frac{(o_{ij} - e_{ij})^{2}}{e_{ij}}}} = \frac{(-56.147)^{2}}{135.147} + \frac{(56.147)^{2}}{91.853} + \frac{(56.147)^{2}}{95.853} + \frac{(-56.147)^{2}}{65.147} = 138.926 $$
We can confirm this computation with the results in the Chi-Square Tests table:
The row of interest here is Pearson Chi-Square and its footnote.
- The value of the test statistic is 138.926.
- Because the crosstabulation is a 2x2 table, the degrees of freedom (df) for the test statistic is $$ df = (R - 1)*(C - 1) = (2 - 1)*(2 - 1) = 1 $$.
- The corresponding p-value of the test statistic is so small that it is cut off from display. Instead of writing "p = 0.000", we instead write the mathematically correct statement p < 0.001.
Since the p-value is less than our chosen significance level α = 0.05, we can reject the null hypothesis, and conclude that there is an association between class rank and whether or not students live on-campus.
- There was a significant association between class rank and living on campus ( Χ 2 (1) = 138.9, p < .001).
- << Previous: Analyzing Data
- Next: Pearson Correlation >>
- Last Updated: May 10, 2024 1:32 PM
- URL: https://libguides.library.kent.edu/SPSS
Street Address
Mailing address, quick links.
- How Are We Doing?
- Student Jobs
Information
- Accessibility
- Emergency Information
- For Our Alumni
- For the Media
- Jobs & Employment
- Life at KSU
- Privacy Statement
- Technology Support
- Website Feedback
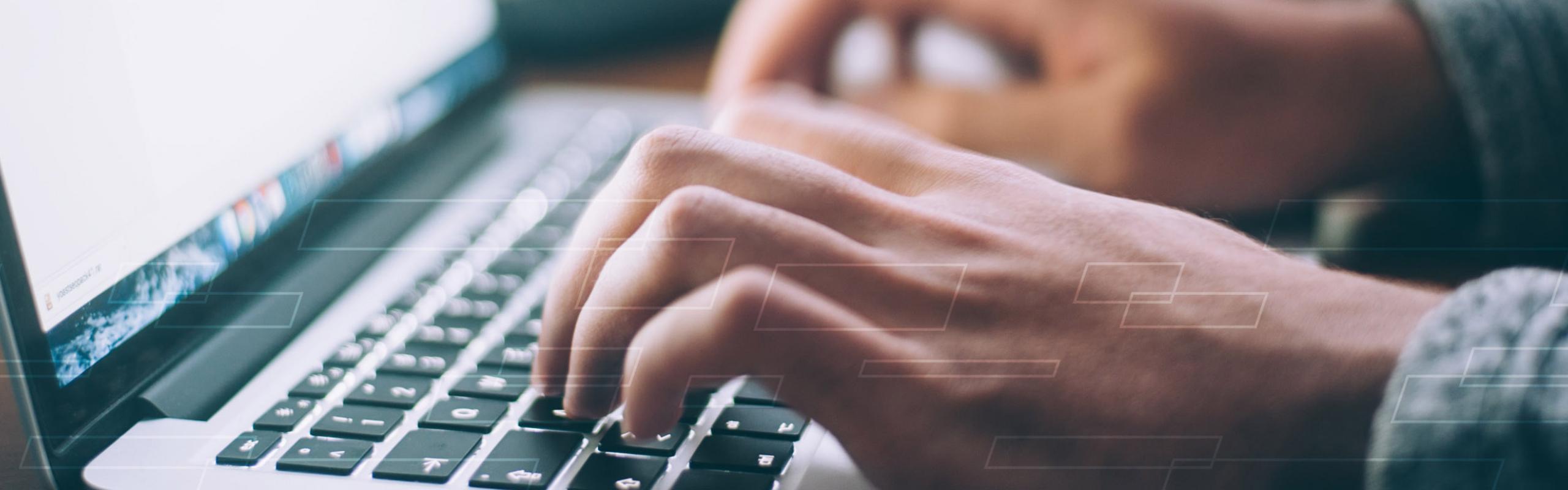
User Preferences
Content preview.
Arcu felis bibendum ut tristique et egestas quis:
- Ut enim ad minim veniam, quis nostrud exercitation ullamco laboris
- Duis aute irure dolor in reprehenderit in voluptate
- Excepteur sint occaecat cupidatat non proident
Keyboard Shortcuts
S.4 chi-square tests, chi-square test of independence section .
Do you remember how to test the independence of two categorical variables? This test is performed by using a Chi-square test of independence.
Recall that we can summarize two categorical variables within a two-way table, also called an r × c contingency table, where r = number of rows, c = number of columns. Our question of interest is “Are the two variables independent?” This question is set up using the following hypothesis statements:
\[E=\frac{\text{row total}\times\text{column total}}{\text{sample size}}\]
We will compare the value of the test statistic to the critical value of \(\chi_{\alpha}^2\) with the degree of freedom = ( r - 1) ( c - 1), and reject the null hypothesis if \(\chi^2 \gt \chi_{\alpha}^2\).
Example S.4.1 Section
Is gender independent of education level? A random sample of 395 people was surveyed and each person was asked to report the highest education level they obtained. The data that resulted from the survey are summarized in the following table:
Question : Are gender and education level dependent at a 5% level of significance? In other words, given the data collected above, is there a relationship between the gender of an individual and the level of education that they have obtained?
Here's the table of expected counts:
So, working this out, \(\chi^2= \dfrac{(60−50.886)^2}{50.886} + \cdots + \dfrac{(57 − 48.132)^2}{48.132} = 8.006\)
The critical value of \(\chi^2\) with 3 degrees of freedom is 7.815. Since 8.006 > 7.815, we reject the null hypothesis and conclude that the education level depends on gender at a 5% level of significance.
If you're seeing this message, it means we're having trouble loading external resources on our website.
If you're behind a web filter, please make sure that the domains *.kastatic.org and *.kasandbox.org are unblocked.
To log in and use all the features of Khan Academy, please enable JavaScript in your browser.
AP®︎/College Statistics
Course: ap®︎/college statistics > unit 12.
- Introduction to the chi-square test for homogeneity
Chi-square test for association (independence)
- Expected counts in chi-squared tests with two-way tables
- Test statistic and P-value in chi-square tests with two-way tables
- Making conclusions in chi-square tests for two-way tables

Want to join the conversation?
- Upvote Button navigates to signup page
- Downvote Button navigates to signup page
- Flag Button navigates to signup page

Video transcript
Chi-Square Calculator
Use this Chi Square calculator to easily test contingency tables of categorical variables for independence or for a goodness-of-fit test. Can be used as a Chi-Square goodness-of-fit calculator , as a Chi-Square test of independence calculator or as a test of homogeneity. Supports unlimited numbers of rows and columns (groups and categories): 2x2, 3x3, 4x4, 5x5, 2x3, 2x4 and arbitrary N x M contingency tables. Outputs Χ 2 and p-value.
Related calculators
- Using the Chi-Square calculator
As a Chi-Square Test of Independence or Homogeneity
As a chi-square test of goodness-of-fit.
- What is a "Chi Squared test"?
- Chi-Square Formula
- Types of Chi-Square tests
Chi-Square Test of Independence
Chi-square test of homogeneity, chi-square goodness-of-fit test, comparing the three types of chi-square tests, other tests, using the chi-square calculator.
The above easy to use tool can function in two main modes: as a goodness-of-fit test and as a test of independence / homogeneity. These modes apply to different situations covered in detail below. The mode of operation can be selected from the radio button below the data input field in the Chi Square calculator interface.
Copy/paste the data from a spreadsheet file into the data input field of the calculator or input it manually by using space ( ) as a column separator and new line as a row separator. The data in all cells should be entered as counts (whole numbers, integers). For example, if you have this data in Excel:
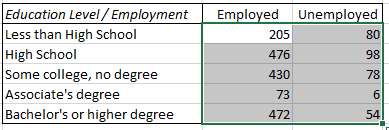
simply copy and paste the numerical cells into the calculator's input field above. Click here to see how this example works. If the sample data is known to be independent the result can be treated as a test of homogeneity. If the data is based on two categorical variables measured from the same population the result can be interpreted as a test of independence between the variables.
Make sure to select the appropriate type of test "Chi-Square test of Goodness-of-fit".
What is a "Chi Squared test"?
A Chi-Squared test is any statistical test in which the sampling distribution of the parameter is Χ 2 -distributed under the null hypothesis and thus refers to a whole host of different kinds of tests that rely on this distribution. In its original version it was developed by Karl Pearson in 1900 as a goodness of fit test: testing whether a particular set of observed data fits a frequency distribution from the Pearson family of distributions (Pearson's Chi-Squared test). Pearson in 1904 expanded its application to a test of independence between the rows and columns of a contingency table of categorical variables [1] . It was further expanded by R. Fisher in 1922-24.
The statistical model behind the tests requires that the variables are the result of simple random sampling and are thus independent and identically distributed (ID) (under the null hypothesis). Consequently, the test can be used as a test for independence or a test for homogeneity (identity of distributions). In certain restricted situations it can also function as a test for the difference in variances. This, however, also means that if one wants to test non-IID data a different test should be chosen.
As with most statistical tests it performs poorly with very low sample size, in particular: because the Χ 2 assumption might not hold well for the data at hand. For a simple 2 by 2 contingency table the requirement is that each cell has a value larger than 5. For larger tables no more than 20% of all cells should have values under 5. Our chi-square calculator will check for some of these conditions and issue warnings where appropriate.
Chi-Square Formula
The formula is the same regardless if you are doing a test of goodness-of-fit, test of independence or of homogeneity . Despite the formula behind all three tests being the same, however, all three have different null hypotheses and interpretations (see below). The Chi-Square formula is simply:
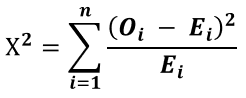
where n is the number of cells in the table and O i and E i are the observed and expected values of each cell. The resulting Χ 2 statistic's cumulative distribution function is calculated from a chi-square distribution with (r - 1) · (c - 1) degrees of freedom (r - number of rows, c - number of columns).
Types of Chi-Square tests
Here we examine the three applications of the Chi Square test: as a test of independence, as a test of homogeneity (identical distribution) and as a goodness-of-fit test.
When using the calculator as a test for independence obtaining a small p-value is to be interpreted as evidence that the two (or more) groups are not independent. Note that if there are more than two variables you cannot say which ones are independent and which are not: it might be all of them or just some of them.
This test refers to testing if two or more variables share the same probability distribution and is also supported by this online Chi Square calculator. The test of homogeneity is used to determine whether two or more independent samples differ in their distributions on a single variable of interest: comparing two or more groups on a categorical outcome. For example, one can compare the educational levels of groups of people from different cities in a country to determine if the proportions between the groups are essentially the same or if there is a statistically significant difference. The null hypothesis H 0 is that the proportions between the groups are the same while the alternative H 1 is that they are different.
Note that upon observing a low p-value one can only say that at least one proportion is different from at least one other proportion, but we cannot say which. Further procedures such as Sheffe, Holm or Dunn-Bonferroni need to be deployed to select a suitable critical value for the further tests to identify pairwise significant differences.
When technically feasible, randomization is often used to produce independent samples.
The goodness-of-fit test can be used to assess how well a certain frequency distribution matches an expected (or known) distribution . The null hypothesis H 0 is that the data follows a specified distribution while the alternative H 1 is that it does not follow that distribution. Rejecting the null means the sample differs from the population on the variable of interest.
For example, if we know that a fair dice should produce each number with a frequency of 1/6 then we can roll a dice 1,000 times, record how many times we observed a given number and then check it against the ideal dice distribution to see if it is fair. If the observations we get are 168 ones, 170 twos, 160 threes, 163 fours, 173 fives and 166 sixes, do we have evidence the dice is rigged? Load example data in the calculator to perform the calculation.
Another example is in population surveys where a representative survey across a certain demographic dimension or geographic locale is required. Knowing the age distribution of the whole population from a recent census or birth & death registries, you can compare the frequencies in your sample to those of the entire population. With a big enough sample the test will be sensitive enough to pick any substantial discrepancy between your sample and the population you are trying to represent.
Yet another application is found in online A/B testing where a Chi-Square goodness-of-fit test is the statistical basis for performing an SRM check . It is used to detect various departures from the assumed statistical model such as randomizer bias, issues with experiment triggering, tracking, log processing, and so on.
This table offers a quick reference to the differences between the three main uses of the Χ 2 test and should be useful to anyone using our X 2 calculator for any purpose.
Under certain conditions the X 2 test can be used as a test for the difference in variances. When both marginal distributions are fixed the Chi-Square test can also be used as a test of unrelated classification.
References
1 Franke T.M. (2012) – "The Chi-Square Test: Often Used and More Often Misinterpreted", American Journal of Evaluation , 33:448 DOI: 10.1177/1098214011426594
Cite this calculator & page
If you'd like to cite this online calculator resource and information as provided on the page, you can use the following citation: Georgiev G.Z., "Chi-Square Calculator" , [online] Available at: https://www.gigacalculator.com/calculators/chi-square-calculator.php URL [Accessed Date: 07 Jun, 2024].
Our statistical calculators have been featured in scientific papers and articles published in high-profile science journals by:
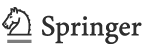
The author of this tool
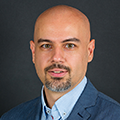
Statistical calculators
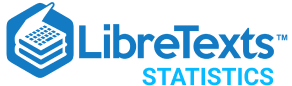
- school Campus Bookshelves
- menu_book Bookshelves
- perm_media Learning Objects
- login Login
- how_to_reg Request Instructor Account
- hub Instructor Commons
Margin Size
- Download Page (PDF)
- Download Full Book (PDF)
- Periodic Table
- Physics Constants
- Scientific Calculator
- Reference & Cite
- Tools expand_more
- Readability
selected template will load here
This action is not available.
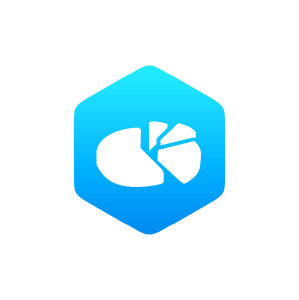
4.3.6: Practice Chi-Square Test of Independence- College Sports
- Last updated
- Save as PDF
- Page ID 22176

- Michelle Oja
- Taft College
\( \newcommand{\vecs}[1]{\overset { \scriptstyle \rightharpoonup} {\mathbf{#1}} } \)
\( \newcommand{\vecd}[1]{\overset{-\!-\!\rightharpoonup}{\vphantom{a}\smash {#1}}} \)
\( \newcommand{\id}{\mathrm{id}}\) \( \newcommand{\Span}{\mathrm{span}}\)
( \newcommand{\kernel}{\mathrm{null}\,}\) \( \newcommand{\range}{\mathrm{range}\,}\)
\( \newcommand{\RealPart}{\mathrm{Re}}\) \( \newcommand{\ImaginaryPart}{\mathrm{Im}}\)
\( \newcommand{\Argument}{\mathrm{Arg}}\) \( \newcommand{\norm}[1]{\| #1 \|}\)
\( \newcommand{\inner}[2]{\langle #1, #2 \rangle}\)
\( \newcommand{\Span}{\mathrm{span}}\)
\( \newcommand{\id}{\mathrm{id}}\)
\( \newcommand{\kernel}{\mathrm{null}\,}\)
\( \newcommand{\range}{\mathrm{range}\,}\)
\( \newcommand{\RealPart}{\mathrm{Re}}\)
\( \newcommand{\ImaginaryPart}{\mathrm{Im}}\)
\( \newcommand{\Argument}{\mathrm{Arg}}\)
\( \newcommand{\norm}[1]{\| #1 \|}\)
\( \newcommand{\Span}{\mathrm{span}}\) \( \newcommand{\AA}{\unicode[.8,0]{x212B}}\)
\( \newcommand{\vectorA}[1]{\vec{#1}} % arrow\)
\( \newcommand{\vectorAt}[1]{\vec{\text{#1}}} % arrow\)
\( \newcommand{\vectorB}[1]{\overset { \scriptstyle \rightharpoonup} {\mathbf{#1}} } \)
\( \newcommand{\vectorC}[1]{\textbf{#1}} \)
\( \newcommand{\vectorD}[1]{\overrightarrow{#1}} \)
\( \newcommand{\vectorDt}[1]{\overrightarrow{\text{#1}}} \)
\( \newcommand{\vectE}[1]{\overset{-\!-\!\rightharpoonup}{\vphantom{a}\smash{\mathbf {#1}}}} \)
We will be using the frequencies of watching college sports and how much college sports teams affect the choice of college attendance again, so here is the contingency table of the Observed frequencies and the table with the Expected frequencies that have been presented before. They are being presented again so that you don't have to go back and forth to find all of the data.
Here's the contingency table with the Observed frequencies:
and the Expected frequencies:
Now we're ready to follow the same 4-step procedure that you've come to know.
Step 1: State the Hypotheses
Chi-Square tests on patterns of relationship, and this doesn't change when we have more than one variable. The research hypothesis does not need to specify how each cell relates to each other like in a factorial ANOVA because we generally don't do pairwise comparisons for Chi-Square analyses. Instead, you can describe a general pattern of relationship between the two variables.
Example \(\PageIndex{1}\)
What is the research hypothesis in words for this scenario? Make sure to describe a general pattern.
- Research hypothesis in words: There will be a pattern of difference such that there will be more people whose college decision was affected by college sports AND who watched college sports.
Remember, the hypotheses in symbols we can only say that the probabilities will not be equal. To determine that, let's figure out what the probability would be if all of the cells were equal. To find that out, we would divide a probability of 100% by the number of cells. There are six cells (Affected Decision=3; Watched=2; \(2\times 3 = 6\)).
\[\dfrac{100}{6} = 16.67 \nonumber \]
So the probability that any random participant will fall into a specific cell is 0.167 for each cell.
Example \(\PageIndex{2}\)
What is the research hypothesis in symbols for this scenario?
- Research hypothesis in symbols: \(P_{EachCell}\neq 0.167\).
If this research hypothesis in symbols doesn't make sense, it might be easier to start with a null hypothesis in words and symbols, then figure out how that works out for the research hypothesis. But honestly, it's not a huge deal if you don't get the probability part of the hypotheses. The important point is that you are testing the null hypothesis that all frequencies will be similar (no pattern of relationship), but that you actually expect a particular pattern (research hypothesis).
Example \(\PageIndex{3}\)
What is the null hypothesis in words and symbols for this scenario?
- Null hypothesis in words: There is no pattern of difference in watching college sports affect college decisions.
- Null hypothesis in symbols: \(P_{EachCell} = 0.167\).
Before we move on to an easier step, let's stop and remind ourselves that, just like correlations, Chi-Square tests cannot show that watching sports caused people to choose their college differently. This scenario is set up to make you think that the IV is watching college sports and the DV is the person's choice of college, but a Chi-Square can't test whether one thing causes another; these statistical analyses can only show if there's a pattern of relationship or not . The design of the experiment (how we collect the data to rule out alternative causes) is how we can show that one variable causes changes in another. This scenario is basically asking the participants if they think that college sports affected their choice of college. It's a small distinction, but an important one.
Step 2: Find the Critical Value
Okay, moving on from the "correlation doesn't equal causation" rant applied to Chi-Square!
Our critical value will come from the same table that we used for the Goodness of Fit Chi-Square test, but our degrees of freedom will change. Because we now have rows and columns (instead of just columns) our new Degrees of Freedom use information on both. This is described at the bottom of the Critical Value of Chi-Square Table page , and looks like this:
- R is the number of rows
- C is the number of columns
What this means is that the number of rows minus one is multiplied by the number of columns minus one. In our example:
\[d f=(2-1)(3-1)=1 \times 2 = 2 \nonumber \]
Exercise \(\PageIndex{1}\)
What is the critical value for this scenario from the p = 0.05 column?
With our df = 2 (\(d f=(2-1)*(3-1)=1 \times 2 = 2 \)), the critical value is 5.991.
Step 3: Calculate the Test Statistic
You probably won't believe it, but you finally have caught a break in learning formulas. The formula for Chi-Square's Goodness of Fit test is the same formula for the Chi-Square Test of Independence!
\[\chi^{2}=\sum_{Each}\left(\dfrac{\left(E-O\right)^{2}}{E} \right)\nonumber \]
If you find a way to combine Table \(\PageIndex{1}\) and Table \(\PageIndex{2}\) with the Differences, Differences Squared and divided by the Expected frequences into one table, then you are a data visualization wizard! For now, we'll create a new table for each step of the formula.
Example \(\PageIndex{4}\)
Use the previous two tables (Table \(\PageIndex{1}\) and Table \(\PageIndex{2}\) ) to create a table of differences by subtracting the Observed frequencies from the Expected frequencies for each cell.
Here is a table of differences:
Notice that the row and column Totals are zero (or nearly so, depending on rounding). This is another good calculation check!
So far, we've accomplished the part in the parentheses of the formula:
What is the next step in this formula?
Square the difference scores in each cell of Table \(\PageIndex{3}\).
The row and column for the Total was removed because those sums aren't used for anything. You can calculate them for completeness, but it won't help you finish the formula. Unless it helps you not get lost!
Now we've finished the numerator of the formula:
What is the next step?
Example \(\PageIndex{5}\)
Using the squared differences in Table \(\PageIndex{4}\):, complete the formula by dividing each cell by it's own Expected frequency (found in Table \(\PageIndex{2}\)). Then, add up all of the Total rows and columns to get the calculated \(\chi^2\).
The Total for summing the Totals for the columns is the same as the sum of the Totals for the rows, so we did it correctly!
Okay, let's see if, for the Test of Independence, doing your calculations in the formula might be easier.
Example \(\PageIndex{6\)
Use the Chi-Square formula to calculate the \(\chi^2\) statistic
Using the information in Table \(\PageIndex{1}\) and Table \(\PageIndex{2}\), we find:
\[\begin{aligned} \chi^{2} &=\dfrac{(35.21-47)^{2}}{35.21}+\dfrac{(25.38-26)^{2}}{25.38}+\dfrac{(26.41-14)^{2}}{26.41}+ \dfrac{(32.79-21)^{2}}{32.79}+\dfrac{(23.62-23)^{2}}{23.62}+\dfrac{(24.59-37)^{2}}{24.59} \end{aligned} \nonumber \]
\[\begin{aligned} \chi_{Diff}^{2} &=\dfrac{(-11.79)^{2}}{35.21}+\dfrac{(-0.62)^{2}}{25.38}+\dfrac{(12.41)^{2}}{26.41}+ \dfrac{(11.79)^{2}}{32.79}+\dfrac{(0.63)^{2}}{23.62}+\dfrac{(-12.41)^{2}}{24.59} \end{aligned} \nonumber \]
\[\begin{aligned} \chi_{Diff Squared}^{2} &=\dfrac{139}{35.21}+\dfrac{0.38}{25.38}+\dfrac{154.01}{26.41}+ \dfrac{139.00}{32.79}+\dfrac{0.40}{23.62}+\dfrac{154.01}{24.59} \end{aligned} \nonumber \]
\[\begin{aligned} \chi_{Division}^{2} &=3.95+0.01+5.83+ 4.24+0.02+6.26 = 20.31 \end{aligned} \nonumber \]
\[ \chi^{2} = 20.31 \nonumber \]
What do you think? Was it easier to do the calculations in five different tables, or do it all in one formula? There's no right answer for this, it really is what's easier for you.
But now, we're ready to make a decision!
Step 4: Make the Decision
What is the final decision?
Exercise \(\PageIndex{2}\)
Should the null hypothesis be retained or rejected?
Our calculated \(\chi^2\)=20.31, and the critical \(\chi^2\) was 5.991, so we would reject the null hypothesis. Our calculated value is so extreme that we would expect it less than 5% of the time if there really was no pattern of relationship between the two qualitative variables.
So what would the statistical sentence look like?
Exercise \(\PageIndex{3}\)
What would our results look like in the statistical sentence?
\(\chi^2\)(2)=20.31, p<.05
Let's use all that we've done to let people know what we found in...
The Write-Up
Can you write this up with the four requirements for reporting results but without descriptive statistics? You can include all of the Observed frequencies, but that gets clunky. A good way around that is to refer to the original table of Observed frequencies.
Example \(\PageIndex{7}\)
Report the results in a concluding paragraph that includes the four requirements.
The research hypothesis was that there will be a pattern of difference such that there will be more people whose college decision was affected by college sports AND who watched college sports A pattern of difference was found (\(\chi^2\)(2)=20.31, p<.05). As can be seen in Table \(\PageIndex{1}\), this research hypothesis was not supported. People who watched college sports seem to believe that they used that to choose their college, and people who didn't watch college sports seem to believe that they did not use college sports to make their decision about which college to choose.
Did you notice all of the "seems like" and "they believe" in that concluding paragraph? Yeah, that's how scientists write. Because science is cumulative, each of one us adds one piece of evidence to a pile that supports one idea. In this case, the idea was that people thought that their choice of college was affected by whether they watched college sports or not. In the Goodness of Fit example, the idea that was supported was that there are about the same amount of people who like and dislike pineapples on pizza. But one study is never conclusive. Instead, many, many scientists conduct many, many studies. Some of them show reality, but some of them (p<.05) find results from their sample that do not match the reality in the population. It can be hard for non-scientists because they might just see us being wishy-washy about our results when we are really following the guidelines of the null hypothesis significance testing procedure.
Let's try one more example so that we've got this Chi-Square thing down.
A computational model for sample dependence in hypothesis testing of genome data
- Original Paper - Cross-Disciplinary Physics and Related Areas of Science and Technology
- Published: 30 May 2024
Cite this article
- Sunhee Kim ORCID: orcid.org/0000-0002-9525-3179 1 &
- Chang-Yong Lee ORCID: orcid.org/0000-0003-1778-6532 1
14 Accesses
Explore all metrics
Statistical hypothesis testing assumes that the samples being analyzed are statistically independent, meaning that the occurrence of one sample does not affect the probability of the occurrence of another. In reality, however, this assumption may not always hold. When samples are not independent, it is important to consider their interdependence when interpreting the results of the hypothesis test. In this study, we address the issue of sample dependence in hypothesis testing by introducing the concept of adjusted sample size. This adjusted sample size provides additional information about the test results, which is particularly useful when samples exhibit dependence. To determine the adjusted sample size, we use the theory of networks to quantify sample dependence and model the variance of network density as a function of sample size. Our approach involves estimating the adjusted sample size by analyzing the variance of the network density, which reflects the degree of sample dependence. Through simulations, we demonstrate that dependent samples yield a higher variance in network density compared to independent samples, validating our method for estimating the adjusted sample size. Furthermore, we apply our proposed method to genomic datasets, estimating the adjusted sample size to effectively account for sample dependence in hypothesis testing. This guides interpreting test results and ensures more accurate data analysis.
This is a preview of subscription content, log in via an institution to check access.
Access this article
Price includes VAT (Russian Federation)
Instant access to the full article PDF.
Rent this article via DeepDyve
Institutional subscriptions

Similar content being viewed by others
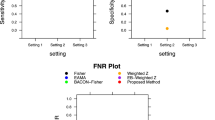
Robust meta-analysis for large-scale genomic experiments based on an empirical approach
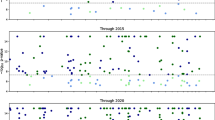
Priors, population sizes, and power in genome-wide hypothesis tests
Control procedures and estimators of the false discovery rate and their application in low-dimensional settings: an empirical investigation, code and script availability.
We have released our analysis tool, a detailed script of the sampling procedure, input files, and output files so that anyone can reproduce the results. These are available at https://github.com/infoLab204/adj_size .
W. Finch, J. Bolin, K. Kelley, Multilevel Modeling Using R (Chapman and Hall/CRC, 2014)
Google Scholar
E. Gómez-de-Mariscal, V. Guerrero, A. Sneider et al., Sci. Rep. 11 , 20942 (2021). https://doi.org/10.1038/s41598-021-00199-5
Article ADS Google Scholar
K. Stewart, Encyclopedia of Quality of Life and Well-Being Research (Springer, New York, 2014), pp.6462–6464
Book Google Scholar
I. Park, H. Lee, Stat. Can. 30 , 183 (2004)
L. Kish, Survey Sampling (John Wiley, New York, 1965)
M. Lin, H. Lucas Jr., G. Shmueli, Inf. Syst. Res. 24 , 906 (2013). https://doi.org/10.1287/isre.2013.0480
Article Google Scholar
A. Barabási, Network Science (Cambridge University Press, Cambridge, 2016)
S. Kim, J. Yun, J. Korean Phys. Soc. 81 , 697 (2022). https://doi.org/10.1007/s40042-022-00590-z
H. Jo, J. Korean Phys. Soc. 82 , 430 (2023). https://doi.org/10.1007/s40042-022-00675-9
R. Nelsen, An Introduction to Copulas (Springer, New York, 1999)
D. Tjøstheim, H. Otneim, B. Støve, Statistical Modeling Using Local Gaussian Approximation (Academic Press, Cambridge, 2021), pp.135–159
D. Lewandowski, D. Kurowicka, H. Joe, J. Multivar. Anal. 100 , 1989 (2009). https://doi.org/10.1016/j.jmva.2009.04.008
K. Zhao, C. Tung et al., Nat. Commun. 13 , 467 (2011). https://doi.org/10.1038/ncomms1467
K. Kim, B. Nawade et al., Front. Plant Sci. 13 , 1036177 (2022). https://doi.org/10.3389/fpls.2022.1036177
K. Zhao, 44K SNP set. (Rice Diversity), http://ricediversity.org/data/index.cfm. Accessed 13 Apr 2024
K. Kim, Data Sheet 1.xlsx (850K_KNU data), https://www.frontiersin.org/articles/10.3389/fpls.2022.1036177/full#supplementary-material. Accessed 13 Apr 2024
W. Qiu, H. Joe, rcorrmatrix (cluster generation) https://rdrr.io/cran/clusterGeneration/man/rcorrmatrix.html. Accessed 13 Apr 2024
W. Press, S. Teukolsky, W. Vetterling, B. Flannery, Numerical Recipes in C: The Art of Scientific Computing , 2nd edn. (Cambridge University Press, Cambridge, 1992), pp.699–706
S. Besenbacher, T. Mailund, M. Schierup, Genetics 181 , 747 (2009). https://doi.org/10.1534/genetics.108.092643
A. Edwards, Genetics 179 , 1143 (2008). https://doi.org/10.1534/genetics.104.92940
G. Di Leo, F. Sardanelli, Eur. Radiol. Exp. 4 , 18 (2020). https://doi.org/10.1186/s41747-020-0145-y
C. Lee, Comput. Biol. Chem. 64 , 94 (2016). https://doi.org/10.1016/j.compbiolchem.2016.06.003
Article MathSciNet Google Scholar
C. Kelley, Iterative Methods for Linear and Nonlinear Equations (SIAM, Philadelphia, 1995)
J. Myers, A. Well, Research Design and Statistical Analysis , 2nd edn. (Lawrence Erlbaum, Mahwah, 2003)
A. Brophy, Behav. Res. Methods 18 , 45 (1986). https://doi.org/10.3758/BF03200993
R-core, cor.test.R (stats package) https://www.rdocumentation.org/packages/stats/versions/3.6.2/topics/cor.test. Accessed 13 Apr 2024
S. Stigler, Ann. Statist. 9 , 465 (1981). https://doi.org/10.1214/aos/1176345451
R. Burden, J. Faires, Numerical Analysis , 9th edn. (Brooks/cole, Pacific Grove, 2010)
Download references
Acknowledgements
We are very grateful to Prof. Yong-Jin Park and Dr. Sang-Ho Chu for providing us with the 580K_KNU datasets. This work was supported by the National Research Foundation of Korea(NRF) grant funded by the Korean government(MSIT) (No. 2022R1A4A1030348), (No. 2021R1I1A3044289), and by the research grant of the Kongju National University in 2021.
Author information
Authors and affiliations.
The Department of Industrial Engineering, Kongju National University, Cheonan, 31080, Republic of Korea
Sunhee Kim & Chang-Yong Lee
You can also search for this author in PubMed Google Scholar
Corresponding author
Correspondence to Chang-Yong Lee .
Additional information
Publisher's note.
Springer Nature remains neutral with regard to jurisdictional claims in published maps and institutional affiliations.
Supplementary Information
Below is the link to the electronic supplementary material.
Supplementary file1 (PDF 217 KB)
Rights and permissions.
Springer Nature or its licensor (e.g. a society or other partner) holds exclusive rights to this article under a publishing agreement with the author(s) or other rightsholder(s); author self-archiving of the accepted manuscript version of this article is solely governed by the terms of such publishing agreement and applicable law.
Reprints and permissions
About this article
Kim, S., Lee, CY. A computational model for sample dependence in hypothesis testing of genome data. J. Korean Phys. Soc. (2024). https://doi.org/10.1007/s40042-024-01100-z
Download citation
Received : 15 April 2024
Revised : 07 May 2024
Accepted : 16 May 2024
Published : 30 May 2024
DOI : https://doi.org/10.1007/s40042-024-01100-z
Share this article
Anyone you share the following link with will be able to read this content:
Sorry, a shareable link is not currently available for this article.
Provided by the Springer Nature SharedIt content-sharing initiative
- Sample dependence
- Hypothesis testing
- Adjusted sample size
- Find a journal
- Publish with us
- Track your research

IMAGES
VIDEO
COMMENTS
A chi-square (Χ 2) test of independence is a nonparametric hypothesis test. You can use it to test whether two categorical variables are related to each other. Example: Chi-square test of independence. Imagine a city wants to encourage more of its residents to recycle their household waste.
A Chi-Square test of independence uses the following null and alternative hypotheses: H0: (null hypothesis) The two variables are independent. H1: (alternative hypothesis) The two variables are not independent. (i.e. they are associated) We use the following formula to calculate the Chi-Square test statistic X2: X2 = Σ (O-E)2 / E.
The Chi-square test of independence determines whether there is a statistically significant relationship between categorical variables.It is a hypothesis test that answers the question—do the values of one categorical variable depend on the value of other categorical variables? This test is also known as the chi-square test of association.
It will be done using the Chi-Square Test of Independence. As with all prior statistical tests we need to define null and alternative hypotheses. Also, as we have learned, the null hypothesis is what is assumed to be true until we have evidence to go against it. In this lesson, we are interested in researching if two categorical variables are ...
The chi-square (\(\chi^2\)) test of independence is used to test for a relationship between two categorical variables. Recall that if two categorical variables are independent, then \(P(A) = P(A \mid B)\). ... As was the case with the single sample and two sample hypothesis tests that you learned earlier this semester, with a large sample size ...
To calculate the chi-squared statistic, take the difference between a pair of observed (O) and expected values (E), square the difference, and divide that squared difference by the expected value. Repeat this process for all cells in your contingency table and sum those values. The resulting value is χ 2.
The Chi-square test of independence checks whether two variables are likely to be related or not. We have counts for two categorical or nominal variables. We also have an idea that the two variables are not related. The test gives us a way to decide if our idea is plausible or not. The sections below discuss what we need for the test, how to do ...
A chi-square test can be used to evaluate the hypothesis that two random variables or factors are independent. This page titled 11.1: Chi-Square Tests for Independence is shared under a CC BY-NC-SA 3.0 license and was authored, remixed, and/or curated by Anonymous via source content that was edited to the style and standards of the LibreTexts ...
The chi-square test is used to determine if there is evidence that the two variables are not independent in the population using the same hypothesis testing logic that we used with one mean, one proportion, etc. Again, we will be using the five step hypothesis testing procedure: 1. Check assumptions and write hypotheses.
A chi-squared test (also chi-square or χ 2 test) ... There are also χ 2 tests for testing the null hypothesis of independence of a pair of random variables based on observations of the pairs. ... then one rejects the null hypothesis of independence. A related issue is a test of homogeneity. Suppose that instead of giving every resident of ...
The test of independence is always right-tailed because of the calculation of the test statistic. If the expected and observed values are not close together, then the test statistic is very large and way out in the right tail of the chi-square curve, as it is in a goodness-of-fit. The number of degrees of freedom for the test of independence is:
We then determine the appropriate test statistic for the hypothesis test. The formula for the test statistic is given below. Test Statistic for Testing H0: p1 = p 10 , p2 = p 20 , ..., pk = p k0. We find the critical value in a table of probabilities for the chi-square distribution with degrees of freedom (df) = k-1.
For the Chi-square, the most commonly used strength test is the Cramer's V test. It is easily calculated with the following formula: χ 2 / n ( k − 1) = χ 2 n ( k − 1) Where n is the number of rows or number of columns, whichever is less. For the example, the V is 0.259 or rounded, 0.26 as calculated below.
Applying the chi-square test for independence to sample data, we compute the degrees of freedom, the expected frequency counts, and the chi-square test statistic. Based on the chi-square statistic and the degrees of freedom, we determine the P-value . DF = (r - 1) * (c - 1) = (2 - 1) * (3 - 1) = 2.
This statistics video tutorial explains how to perform a hypothesis test of independence using the chi-square distribution.Confidence Intervals & Margin of E...
Step 2: Perform the Chi-Square Test of Independence. Next, we can perform the Chi-Square Test of Independence using the chisq.test () function: #Perform Chi-Square Test of Independence. chisq.test(data) Pearson's Chi-squared test. data: data. X-squared = 0.86404, df = 2, p-value = 0.6492. The way to interpret the output is as follows: Chi ...
Chi-Square Test of Independence. The Chi-Square Test of Independence determines whether there is an association between categorical variables (i.e., whether the variables are independent or related). It is a nonparametric test. This test is also known as: Chi-Square Test of Association. This test utilizes a contingency table to analyze the data.
This test is performed by using a Chi-square test of independence. Recall that we can summarize two categorical variables within a two-way table, also called an r × c contingency table, where r = number of rows, c = number of columns. Our question of interest is "Are the two variables independent?".
Hypothesis testing: Hypothesis testing for the chi-square test of independence as it is for other tests like ANOVA, where a test statistic is computed and compared to a critical value. The critical value for the chi-square statistic is determined by the level of significance (typically .05) and the degrees of freedom.
To meet the condition of Large counts for any X^2 Statistic. When specifically does one use a T-test and a chi-square test. A t-test is used to determine the difference between two sets of data. A chi-square test involves looking for a relationship (homogeneity, independence, or goodness-of-fit.)
To perform a Chi-Square Test of Independence, simply fill in the cells below for a contingency table of up to 5 rows and 5 columns. If your table is smaller than 5×5, simply leave the other cells blank. X 2 Test Statistic: 0.864035. p-value: 0.649198.
For a test with more than \(2\) rows or columns, use G*Power to calculate the sample size needed for a test of independence. Under Test Family, choose chi-square tests, and under Statistical Test, choose Goodness-of-Fit Tests: Contingency Tables. Under Type of Power Analysis, choose A Priori: Compute Required Sample Size.
Versatile Chi square test calculator: can be used as a Chi square test of independence calculator or a Chi square goodness-of-fit calculator as well as a test for homogeneity. Supports unlitmited N x M contingency tables: 2 by 2 (2x2), 3 by 3 (3x3), 4 by 4 (4x4), 5 by 5 (5x5) and so on, also 2 by 3 (2x3) etc with categorical variables. Chi square goodness-of-fit calculator online.
The formula for Chi-Square's Goodness of Fit test is the same formula for the Chi-Square Test of Independence! χ2 = ∑Each((E − O)2 E) χ 2 = ∑ E a c h ( ( E − O) 2 E) If you find a way to combine Table 4.3.6.1 4.3.6. 1 and Table 4.3.6.2 4.3.6. 2 with the Differences, Differences Squared and divided by the Expected frequences into one ...
Statistical hypothesis testing assumes that the samples being analyzed are statistically independent, meaning that the occurrence of one sample does not affect the probability of the occurrence of another. ... we performed a \(\chi ^{2}\) test of independence between SNP genotypes and phenotypes in the samples. Fig. 5. The plot of the variance ...